Importance of dynamic aortic evaluation in planning TEVAR
Introduction
The biomechanics of the thoracic aorta play an essential role in the endovascular treatment of the descending thoracic aorta and its side branches. The pulsatility of the aorta and its side branches during the cardiac cycle, the heartbeat-dependent out-of-plane movement of the aorta, and other morphologic factors might all be of influence on the endovascular treatment for thoracic aortic pathology (1). When patients affected by thoracic aortic diseases are selected for thoracic endovascular aortic repair (TEVAR), preoperative assessment of aortic morphology is essential. In particular, the aortic arch angulation and the proximal and distal landing zones are important in order to achieve an optimal result. Adequate sizing in patients with acute type B aortic dissection (ABAD) is challenging, because the actual size of the affected true lumen is unknown and the aortic wall is expected to be more fragile than in patients with thoracic aortic aneurysm (TAA). Moreover, a stent graft in a patient with ABAD will most likely have sealing over the entire length, while in an aneurysm the stent graft has only proximal and distal sealing and fixation zones. Therefore, moderate stent graft oversizing, less radial force of the stent graft and the absence of anchoring pins should be considered when planning TEVAR for ABAD.
Computed tomography angiography (CTA) is most commonly used to analyze the aorta and the surrounding structures, while magnetic resonance angiography (MRA) is usually adopted as second choice. Generally, using CTA, static images are acquired, and these images have been obtained at any arbitrary point during the cardiac cycle (during diastole, during systole or in between). Dynamic electrocardiographic-gated (ECG-gated) CTA, MRA and ultrasonography imaging have demonstrated significant changes in aortic dimensions during the cardiac cycle (2,3). Irrespective of the imaging modalities, static imaging protocols do not take normal aortic dynamics into account and may subsequently lead to incorrect measurements. Guidelines state that a stent graft should be 10-20% oversized in comparison to the aortic diameter (4). However, a maximum aortic difference in pulsatility has been reported around 18% in the ascending and descending thoracic aorta (5,6) (Figures 1,2), and therefore the use of static imaging for TEVAR planning can lead to relative undersizing of the stent graft to the aortic diameter. This potential mismatch might be the reason for common stent graft related complications, such as migration and endoleak type I (1,3). Distensibility of the thoracic aorta is maintained after TEVAR, which makes adequate sizing even more crucial (7). The integration of dynamic imaging into clinical practice, as a standard approach, can lead to better stent graft sizing and subsequently an improved outcome (8). Additionally, it can provide insight into the causative mechanisms for stent graft related complications and improve stent graft performance.
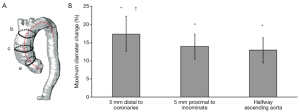
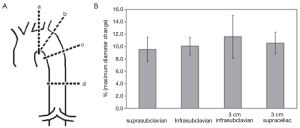
Advances in medical imaging
Over the past two decades, vascular medical imaging has progressed extensively, with the introduction of new imaging modalities considering aortic hemodynamics. ECG-gated CT and ECG-gated MRI both permit studying the pulsatility of the aorta and surrounding tissue during the cardiac cycle, and allow insight into aortic morphologic changes.
ECG-gated CT and Dual-energy CT
ECG-gated CT allows studying the pulsatility of the aorta and stent graft, by obtaining images at different time points during the cardiac cycle. ECG synchronization with a 16 to 256 slices CT scanner is mandatory for such scans, with more slices resulting in lower temporal solution, and congruently in less radiation exposure and contrast administration (9). A minimum temporal resolution of 8 phases per cardiac cycle can offer an adequate perspective of the aortic dynamics. Moreover, all major vendors have introduced iterative reconstruction algorithms for CT, which are noise-reducing methods that aim to either enhance image quality using a constant CT radiation dose, or to reduce the CT radiation dose with similar image quality (10). Dual-energy CT (DECT) imaging is a relatively new technique using two different X-ray tubes in a single CT unit, which discriminates materials based on diverse interactions with photons between the different X-ray energies. In the near future, this technique may be further implemented into clinical practice for the evaluation of aortic conditions. In particular, DECT can be used to remove bones from the datasets and virtual images allow differentiation between the contrast agent, and calcifying thrombus in the thoracic aorta of endovascularly-treated patients (11).
ECG-gated MRI
ECG-gated MRI, similar to ECG-gated CT, enables study of the pulsatility of the aorta and stent graft, by obtaining a sequence of images throughout the cardiac cycle and combining the MR images with an ECG. These images contain three spatial dimensions and one temporal solution, which is the time between the subsequent images. Compared to CTA, MR imaging is considered safer, as no radiation exposure is necessary for this high-resolution imaging modality. However, the processing time is relatively long and it is vital that patients do not move during the scan for optimal results. ECG-gated MRI is frequently used for cardiac examination and can also be used to assess the aortic motion in the preoperative setting for optimal stent graft sizing in patients with thoracic aortic pathology.
Besides stent graft sizing, dynamic MRA may also be useful for other purposes in the preoperative examination including preservation of the artery that provides the main blood supply of the anterior spinal artery (i.e., artery of Adamkiewicz), which is important to reduce the risk of spinal cord ischemia after TEVAR (12). Dynamic MRA has shown high sensitivity in detecting this artery, and subsequently the endovascular procedure can be adjusted to provide maximal spinal cord protection. Dynamic MRA is also adopted for postoperative evaluation, and, compared to conventional angiography, it has been shown to be an excellent imaging modality for the classification of endoleaks after endovascular aortic repair (13,14).
An additional dynamic application of MRI is the flow study with phase contrast sequence to assess the peak velocity, forward and reverse flow, and visualization of flow. The raw MR signal consists of two components: a magnitude and a phase. The magnitude holds anatomic information, and the phase holds velocity information, in addition to various background sources (15).
4D PC-MRI
An imaging modality with the potential to be widely implemented into clinical practice is four-dimensional phase-contrast magnetic resonance imaging (4D PC-MRI) (16,17). This imaging technique can be used for in vivo imaging of the blood flow within the cardiovascular system with several potential clinical applications. Volumetric flow measurements, such as cardiac output and valvular regurgitation, are already used in clinical practice, and other applications will undoubtedly follow. Coronary flow assessment to evaluate coronary artery disease, as well as aortic flow evaluation in patients with dissection and other applications, such as blood flow imaging in peripheral arteries and neurovascular systems, are of potential clinical use (18-20).
In patients with aortic dissection, 4D PC-MRI can accurately visualize and quantify flow characteristics and provide valuable information about stroke volume, velocity, dominant proximal and distal entry tears and helical flow, which seem all related to aortic expansion (Figure 3) (16). The velocity is measured in three directions encoded in 3D imaging over time, therefore enabling extensive analysis of physiologic hemodynamics in vivo. Four-dimensional PC-MRI is currently only available in some specialized centers, but has the potential to be widely adopted, because no ionizing radiation is used. With MRI scanners becoming more common in the clinical setting and continuously improving technology (i.e., accelerated acquisition, imaging hardware), the application of velocity imaging with MRI will also continue to grow. Additionally, imaging technologists and clinicians are collaborating and also developing visualization and quantitative tools to streamline the large amount of information obtained in the complex velocity fields.
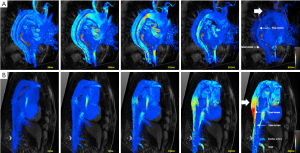
Computational fluid dynamics (CFD) and TEVAR
In recent years, an increasing number of studies focused on aortic hemodynamics after TEVAR using CFD, which is a numerical technique for evaluating the hemodynamic environment of a vessel segment (21-23). Phase contrast and MRA have been extensively used in the CFD field, providing flow boundary conditions in which numerical simulations can be performed and represent a method of validating of calculated flow fields (24,25).
A patient-specific approach to both stent graft design and procedural approach is required to distinguish between the pathologic and the healthy aortic condition. To successfully accomplish this goal, integration of knowledge between different scientific fields is required (i.e., engineering, informatics and medicine). The technological development in medical imaging and parallel computing has contributed to the ability to perform complex simulations for patient-specific modeling. These simulations might be used and further implemented into clinical decision-making in the near future (26).
A patient-specific computational model can be created, using information from medical imaging, to investigate the different forces acting on the aortic wall and stent graft (21). In addition, CFD can help in understanding the magnitude and orientation of the loads experienced by thoracic aortic stent grafts in vivo, with the aim of improving stent graft design and performance (27). Furthermore, the different hemodynamic and biomechanical forces acting on the intermodular junctions of a multi-component stent graft can be investigated through computational modeling, focusing on the development of endoleak due to disconnection of the stent graft segments (22). Moreover, CFD can depict the flow conditions and the magnitude of flow by streamlines throughout the thoracic aorta (Figure 4) (28).
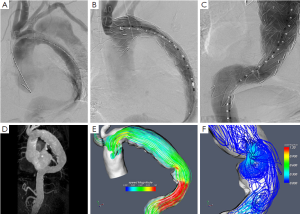
Other applications for TEVAR
TEVAR has proven to be beneficial over open surgical repair, both in TAA and ABAD, and is currently the preferred therapy in these patients. Previous studies have shown that there is significant distension of the thoracic (descending) aorta, both pre- and post TEVAR, during the cardiac cycle in patients with TAA (7). Most interestingly, distension was preserved after stent graft placement. Based on these conditions, it has to be concluded that aortic dynamics have a profound impact on correct stent graft sizing, design and durability (7).
The primary goal of TEVAR for ABAD is to cover the primary entry tear. However, due to possible uncovered re-entry tears, communication between the true and false lumen can still be present postoperatively, inhibiting the false lumen thrombosis and aortic remodeling process. By using preoperative computational flow analyses, it is possible to detect and quantify the importance of these re-entry tears/multiple entry tears, potentially assisting the physician in the choice of endovascular procedure and its extent (29).
Current status of dynamic aortic evaluation and guidelines for future research
Currently, numerous relative new dynamic imaging applications (i.e., ECG-gated CT and MRI, 4D PC-MRI, CFD analysis) have been reported in the literature. This development has expanded the multidisciplinary integrated knowledge and evaluation of the diseased thoracic aorta. However, several issues concerning the aortic arch biomechanics and their clinical consequences remain undefined and should be investigated in future research.
Larger prospective studies are required to measure flow characteristics and clinical outcomes in preoperative planning for TEVAR and in the postoperative phase. The large load of quantitative information needs to be further streamlined to be able to widely implement different new dynamic imaging tools and modalities into daily clinical practice. This will allow physicians to further determine the predictive value of the dynamic measurements. More specifically, in stratifying patients with ABAD, a new imaging modality such as 4D PC-MRI may have predictive value.
Conclusions
Technological development and interdisciplinary collaboration provide the opportunity to progressively implement dynamic imaging for aortic evaluation in planning TEVAR into clinical practice. Dynamic imaging can help preoperatively in accurate stent graft selection and sizing, and postoperatively to evaluate stent graft performance. Important imaging modalities, such as 4D PC-MRI, can give valuable information about adequate sizing and the presence and potential coverage of entry tears. Eventually, further implementation of (new) dynamic imaging tools will lead to a better outcome for the patient, which is the bottom line of research in this field.
Acknowledgements
Prof. F. Auricchio and Dr. M. Conti acknowledge the support of Ministero dell’Istruzione, dell’Università e della Ricerca through the Project no. 2010BFXRHS; ERC Starting Grant through the Project ISOBIO: Isogeometric Methods for Biomechanics (No. 259229).
Disclosure: The authors declare no conflict of interest.
References
- van Keulen JW, van Prehn J, Prokop M, et al. Dynamics of the aorta before and after endovascular aneurysm repair: a systematic review. Eur J Vasc Endovasc Surg 2009;38:586-96. [PubMed]
- Laskowski I, Verhagen HJ, Gagne PJ, et al. Current state of dynamic imaging in endovascular aortic aneurysm repair. J Endovasc Ther 2007;14:807-12. [PubMed]
- van Herwaarden JA, Bartels LW, Muhs BE, et al. Dynamic magnetic resonance angiography of the aneurysm neck: conformational changes during the cardiac cycle with possible consequences for endograft sizing and future design. J Vasc Surg 2006;44:22-8. [PubMed]
- van Prehn J, Schlösser FJ, Muhs BE, et al. Oversizing of aortic stent grafts for abdominal aneurysm repair: a systematic review of the benefits and risks. Eur J Vasc Endovasc Surg 2009;38:42-53. [PubMed]
- Muhs BE, Vincken KL, van Prehn J, et al. Dynamic cine-CT angiography for the evaluation of the thoracic aorta; insight in dynamic changes with implications for thoracic endograft treatment. Eur J Vasc Endovasc Surg 2006;32:532-6. [PubMed]
- van Prehn J, Vincken KL, Muhs BE, et al. Toward endografting of the ascending aorta: insight into dynamics using dynamic cine-CTA. J Endovasc Ther 2007;14:551-60. [PubMed]
- van Prehn J, Bartels LW, Mestres G, et al. Dynamic aortic changes in patients with thoracic aortic aneurysms evaluated with electrocardiography-triggered computed tomographic angiography before and after thoracic endovascular aneurysm repair: preliminary results. Ann Vasc Surg 2009;23:291-7. [PubMed]
- van Bogerijen GHW, Tolenaar JL, Conti M, et al. Contemporary Role of Computational Analysis in Endovascular Treatment for Thoracic Aortic Disease. Aorta 2013;1:171-81.
- Sabarudin A, Sun Z, Ng KH. A systematic review of radiation dose associated with different generations of multidetector CT coronary angiography. J Med Imaging Radiat Oncol 2012;56:5-17. [PubMed]
- Willemink MJ, Habets J, de Jong PA, et al. Iterative reconstruction improves evaluation of native aortic and mitral valves by retrospectively ECG-gated thoracoabdominal CTA. Eur Radiol 2013;23:968-74. [PubMed]
- Ko JP, Brandman S, Stember J, et al. Dual-energy computed tomography: concepts, performance, and thoracic applications. J Thorac Imaging 2012;27:7-22. [PubMed]
- Hyodoh H, Kawaharada N, Akiba H, et al. Usefulness of preoperative detection of artery of Adamkiewicz with dynamic contrast-enhanced MR angiography. Radiology 2005;236:1004-9. [PubMed]
- Lookstein RA, Goldman J, Pukin L, et al. Time-resolved magnetic resonance angiography as a noninvasive method to characterize endoleaks: initial results compared with conventional angiography. J Vasc Surg 2004;39:27-33. [PubMed]
- van der Laan MJ, Bakker CJ, Blankensteijn JD, et al. Dynamic CE-MRA for endoleak classification after endovascular aneurysm repair. Eur J Vasc Endovasc Surg 2006;31:130-5. [PubMed]
- Thompson RB, McVeigh ER. High temporal resolution phase contrast MRI with multiecho acquisitions. Magn Reson Med 2002;47:499-512. [PubMed]
- Clough RE, Waltham M, Giese D, et al. A new imaging method for assessment of aortic dissection using four-dimensional phase contrast magnetic resonance imaging. J Vasc Surg 2012;55:914-23. [PubMed]
- Frydrychowicz A, François CJ, Turski PA. Four-dimensional phase contrast magnetic resonance angiography: potential clinical applications. Eur J Radiol 2011;80:24-35. [PubMed]
- de Boorder MJ, Hendrikse J, van der Grond J. Phase-contrast magnetic resonance imaging measurements of cerebral autoregulation with a breath-hold challenge: a feasibility study. Stroke 2004;35:1350-4. [PubMed]
- Nitz WR, Bradley WG Jr, Watanabe AS, et al. Flow dynamics of cerebrospinal fluid: assessment with phase-contrast velocity MR imaging performed with retrospective cardiac gating. Radiology 1992;183:395-405. [PubMed]
- Rutgers DR, Blankensteijn JD, van der Grond J. Preoperative MRA flow quantification in CEA patients: flow differences between patients who develop cerebral ischemia and patients who do not develop cerebral ischemia during cross-clamping of the carotid artery. Stroke 2000;31:3021-8. [PubMed]
- Lam SK, Fung GS, Cheng SW, et al. A computational study on the biomechanical factors related to stent-graft models in the thoracic aorta. Med Biol Eng Comput 2008;46:1129-38. [PubMed]
- Prasad A, To LK, Gorrepati ML, et al. Computational analysis of stresses acting on intermodular junctions in thoracic aortic endografts. J Endovasc Ther 2011;18:559-68. [PubMed]
- Tse KM, Chiu P, Lee HP, et al. Investigation of hemodynamics in the development of dissecting aneurysm within patient-specific dissecting aneurismal aortas using computational fluid dynamics (CFD) simulations. J Biomech 2011;44:827-36. [PubMed]
- Saber NR, Wood NB, Gosman AD, et al. Progress towards patient-specific computational flow modeling of the left heart via combination of magnetic resonance imaging with computational fluid dynamics. Ann Biomed Eng 2003;31:42-52. [PubMed]
- Wood NB, Weston SJ, Kilner PJ, et al. Combined MR imaging and CFD simulation of flow in the human descending aorta. J Magn Reson Imaging 2001;13:699-713. [PubMed]
- Taylor CA, Figueroa CA. Patient-specific modeling of cardiovascular mechanics. Annu Rev Biomed Eng 2009;11:109-34. [PubMed]
- Figueroa CA, Taylor CA, Chiou AJ, et al. Magnitude and direction of pulsatile displacement forces acting on thoracic aortic endografts. J Endovasc Ther 2009;16:350-8. [PubMed]
- Midulla M, Moreno R, Baali A, et al. Haemodynamic imaging of thoracic stent-grafts by computational fluid dynamics (CFD): presentation of a patient-specific method combining magnetic resonance imaging and numerical simulations. Eur Radiol 2012;22:2094-102. [PubMed]
- Chen D, Müller-Eschner M, Kotelis D, et al. A longitudinal study of Type-B aortic dissection and endovascular repair scenarios: computational analyses. Med Eng Phys 2013;35:1321-30. [PubMed]