Arterial grafts: clinical classification and pharmacological management
Introduction
Since the early days of coronary artery bypass grafting (CABG), the biological characteristics and the differences between venous and arterial grafts had already attracted research interest. There is a large body of literature, including our own work, which have demonstrated differences between venous and arterial grafts. For example, the use of the internal mammary artery (IMA) has proven to provide superior long-term results than standard saphenous vein grafts (1,2). Some of the differences between the two types of conduits, which may account for the variations in the long-term patency rate, include:
- Veins are more susceptible to vasoactive substances than arteries (3);
- The venous wall is supplied by the vasa vasorum whereas the arterial wall may be supplied through the lumen in addition to the vasa vasorum (4);
- The endothelium of arteries may secrete more endothelium-derived relaxing factor (EDRF) (5) and may release more nitric oxide (NO) (6,7) and endothelium-derived hyperpolarizing factor (EDHF) (6,7);
- The structure of the vein is subject to low pressure whereas that of the artery is subject to high pressure. After grafting to the aorta-coronary system, venous grafts have to adapt to the higher pressure.
We will describe the biological characteristics of arterial and venous grafts, clinical classification, and scientific considerations for clinical choice of arterial grafts and pharmacological management with antispasm protocols for conduits.
Arterial grafts
Based on the superior long-term results of the IMA, other arteries have been used for CABG (8-14). These conduits include the radial artery (RA) (8), the gastro-epiploic artery (GEA) (9), the inferior epigastric artery (IEA) (10,11), the splenic artery (12), the subscapular artery (13), the inferior mesenteric artery (14), the descending branch of lateral femoral circumflex artery (15), and the ulnar artery (16). In addition, the intercostal artery (17) has also been suggested for use as a graft.
The long-term patency rates for IMA are well established. Similarly, long-term patency for RA (18-23) and GEA (24-27) are also now well recognized, supplementing early reports (28-30). Other arterial conduits are expected to exhibit similar long-term results as the IMA, as it is hypothesized that all arterial conduits have similar biological characteristics such as contractility, relaxing characteristics, endothelial function, and anatomical structure.
However, histological studies have revealed that there are major variations between various grafts in terms of the structure of smooth muscle, such as elastic lamellae (4,31), while comparative functional studies have demonstrated that there are differences with regard to contractility and endothelial function (32-36). Our previous studies have demonstrated that the endothelium of the IMA releases more NO and EDHF than the RA at both the basal and stimulated level (37) and we have recently further shown that the expression and function of endothelial nitric oxide synthase messenger RNA and protein are higher in the IMA than in the RA (38).
These anatomical and physiological dissimilarities form the basis for the divergent clinical manifestations, postoperative functions, and long-term patency rates for the various grafts. Furthermore, it has been observed that the tendency for differing arterial grafts to develop spasms during surgical dissection and perioperative period differs. It is the experience of many surgeons that the GEA has a higher tendency to spasm than the IMA (39). Similarly, in the early adoption of RA grafts in the 1970s, spasm of the RA was a sufficiently serious problem that, together with a low patency rate, led to the abandonment of this arterial graft for clinical use (25). Only after the development of a method to overcome spasm of this arterial graft was it then used again (40,41).
Biological characteristics
A common feature of arterial grafts is that removal of these arteries would not usually affect the blood supply to the organ, as these are conductance arteries and will only be a concern under extreme circumstances. However, there may be differences in the function of these arteries due to different flow and capacitance requirements of their native organ. The differences among these arteries can be described from anatomical, physiological, pharmacological, and embryological perspectives at the organ, tissue, or cellular/molecular level.
Anatomy
The differences in the gross anatomy of the arterial grafts are obvious since they are at different locations in the body and supply different organs. In addition to evidence showing the divergent structure of arteries (4,31), another structural difference is that some arteries such as GEA, IEA and RA contain more smooth muscle cells in their wall and therefore are less elastic. In contrast, other arteries such as IMA may be more elastic and contain higher levels of elastic laminae. These structural variations may account for the differences in the physiological and pharmacological reactivity of the conduits.
Contractility and incidence of spasm
While the true cause of vasospasm remains unclear, vasospasm is presumed to be the extreme form of vasoconstriction responses to stimuli (spasmogens). These stimuli may be physical (such as mechanical stimulation or temperature changes) or pharmacological (such as nerve stimulation or vasoconstrictor substances) (35,42-57).
We have suggested (35) that there are two types of vasoconstrictors that are important spasmogens for arterial grafts. Type I [endothelin, prostanoids (TxA2 and PGF2α) and α1-adrenoceptor agonists] are the most potent vasoconstrictors which strongly contract arterial grafts even when the endothelium is intact, while Type II vasoconstrictors (such as 5-HT) only induce a weak vasoconstriction when the endothelium is intact. However, these vasoconstrictors probably play an important role in the spasm of arterial grafts if the endothelium is lost due to surgical handling or from diseases such as diabetes.
Although all arterial grafts react to the vasoconstrictors listed above, there is a general trend that some arteries react to vasoconstrictors in a stronger manner than others (33,34,39,41,48). Despite these variations, there are groups of arteries, including IMA and IEA, that are similar in their contractility response to vasoconstrictors such as endothelin, U46619, or K+ (34,36).
We have also compared the pharmacological reactivity of the human coronary artery from the explanted heart transplant with the bypass grafts in vitro (34). When the large coronary artery has atherosclerotic disease, it may be less reactive to vasoconstrictors compared to arterial grafts, even though the reactivity of the micro-coronary artery may remain high.
Receptors in smooth muscle
Most vasoconstrictors, except potassium ions, contract arterial grafts by activating a specific receptor. For example, the IMA is an α1-adrenoceptor-dominant artery with little α2- or β-function (49,58), in contrast to the RA which has both α1- and α2-function but weak β-function (50).
ETA, ETB (51), 5-HT (52), angiotensin (53), thromboxane-prostanoid (TP) (54), vasopressin V1 receptors (44,55), and vasoactive intestinal peptide (45) receptors have been demonstrated to be functional in the IMA. However, there have been fewer reports on the receptors in other arterial grafts (34,45,48). Moreover, we have recently discovered that the human urotensin II receptor (hUT receptor) exists in the human IMA (46,47) and RA (46).
Receptors in endothelium and endothelial function
Receptors are also located in the cellular membrane of the endothelial cell in the arterial grafts. For example, common stimuli for EDRF such as acetylcholine, bradykinin, and substance P are present in the endothelium of arterial grafts (32,36,48). The vascular endothelial growth factor (VEGF)-induced, endothelium-dependent relaxation, mediated by both NO and prostacyclin in the IMA, has been shown mainly through the KDR receptors, rather than Flt-1 receptors (59). Most recently, we have shown that corticotropin-releasing factor (CRF) receptors CRF1, CRF2α, and CRF2β are present in the human IMA (60). The CRF urocortin-induced endothelium-dependent relaxation in the IMA is likely to be mediated through CRF receptors situated in the endothelium of the IMA (60).
In our recent studies comparing endothelial function among arterial grafts, it was found that IMA has more endothelium-dependent relaxation than the IEA in response to acetylcholine and calcium ionophore A23187 (36), although it is still uncertain whether this is due to intrinsic physiological differences or due to the higher incidence of atherosclerosis in the IEA (31,36). In addition, others also found differences in endothelial function among arterial grafts (56,57).
Most importantly, we have previously demonstrated that the IMA releases more NO and has greater EDHF-mediated hyperpolarisation compared to the saphenous vein (6) and the RA (37). NO and EDHF are the two major EDRFs in arteries (61,62). The higher expression of endothelial NO synthase (eNOS) in the IMA relative to the RA may explain the higher release of NO in IMA (38). These direct and quantitative studies reveal that the IMA has superior endothelial function due to its intrinsic characteristics and this is closely related to its excellent long-term patency.
Smooth muscle relaxation
No major differences have been observed among arterial grafts in the endothelium-independent relaxation (such as to nitroglycerine) (32,36) although there may be some differences in response to vasodilator substances with regard to sensitivity (45).
Embryological considerations
Commonly used arterial grafts belong to different groups of arteries in various locations of the body and can be divided into somatic arteries and splanchnic arteries (63). Somatic arteries supply the body wall and include the IMA, the IEA, the subscapular artery and the intercostal artery, while splanchnic arteries supply visceral organs and include the GEA and the splenic artery. Embryonic studies (63) have shown that somatic arteries develop from intersegmental branches to the body wall whereas splanchnic arteries develop from segmental branches of primitive dorsal aorta to supply the digestive tube.
Arteries that supply extremities and limb arteries belong to a special type. Upper limb arteries are developed from somatic arteries whereas lower limb arteries develop from the dorsal root of the umbilical artery.
Physiological considerations
Arterial grafts for coronary surgery are conduit arteries which function to carry blood flow to organs. Since the arterial grafts supply organs with different physiological functions, these arteries have differences in structure and reactivity in order to adapt to the need for blood supply to those individual organs. This explains why some of them are more readily spastic (more reactive to vasoconstrictors) than others.
Segmental difference
The reactivity of the grafts varies along the length of conduit arteries, as evident in the IMA where the mid portion is less reactive compared to the distal and the proximal portions (64,65). The major muscular components are located at the two ends of the artery (muscular regulator), which may also be true for other arterial grafts (66). In particular, the distal end is more efficient as the physiological regulator for flow because this region contains relatively more smooth muscle cells and is smaller in diameter, characteristics which are important for regulating distribution of blood flow. Paradoxically, these characteristics may also be detrimental when such arteries are used as bypass grafts. In terms of preventing vasospasm of the arterial grafts, trimming off the small and highly reactive distal end of the grafts may be important and clinically feasible (64).
Incidence of atherosclerosis
In general, the incidence of atherosclerosis in the four major arterial grafts is low compared with the left anterior descending artery (LAD) (4). Particularly, early studies demonstrated low incidence of atherosclerosis in the IMA (67) through angiograms, which also frequently show that a patent IMA exists with a stenotic vertebral artery. In contrast, the incidence of atherosclerosis at the proximal end of the IEA may be high as seen in a small group of patients (31,36). This may be related to the high incidence of atherosclerosis in the lower limb than the upper limb arteries and that the IEA is the first branch of the external iliac artery (36). The incidence of atherosclerosis is low in the GEA (31,68). In the IMA grafts (1,2), the incidence of atherosclerosis is still low (69) even 15 to 21 years later. There is evidence showing that it could be also low in the RA (70).
Pharmacology of vasoactive substances in arterial grafts
Arterial grafts are conductance arteries which react with vasoactive substances such as vasoconstrictor substances and vasodilator substances. Since this is a large topic it is impossible to include in this article, the reader may find more details in reference 71 that cover this issue. Our original studies on this topic are also recommended for reading (42-44,46-50,53,54,59,71-90,91-113).
Clinical classification and selection
The variations of arterial grafts’ biological characteristics should be taken into account when selecting appropriate use of such grafts. To better understand the biological behavior of the grafts, their common features and the differences, a clinical classification may be useful for the practicing surgeon.
Clinical classification
Based on experimental studies on their vasoreactivity, taken together with the anatomical, physiological, and embryological considerations described above, we have proposed a functional classification for arterial grafts that may be useful clinically (34,114) (Figure 1).
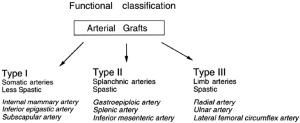
Our classification suggests that there are three types of arterial grafts as follows:
- Type I, somatic arteries;
- Type II, splanchnic arteries;
- Type III, limb arteries.
From anatomical considerations, somatic arteries (Type I) such as the IMA are located in and supply blood to the body wall. Additionally, other somatic arteries such as the IEA, the subscapular artery, or the intercostal artery have similar contractility to the IMA. This has already been demonstrated for the IEA (4), although there are no data available yet for the others. While the IEA was histologically demonstrated as a muscular artery (4), its pharmacological reactivity (34) as well as embryonic origin is similar to the IMA. Furthermore, the wall of the IEA is thinner than the GEA (46) and therefore we classified this artery as Type I, together with the IMA.
Splanchnic (visceral) arteries (Type II) such as the GEA supply blood to visceral organs. Other splanchnic arteries, including the splenic artery and the inferior mesenteric artery, have similar reactivity to the GEA although this requires experimental confirmation. Type III arteries such as the RA are located in the limb. Other limb arteries such as the ulnar artery and the lateral femoral circumflex artery are also in this group.
As previously mentioned, the Type II artery GEA and the Type III artery RA have higher pharmacological reactivity to vasoconstrictors, a characteristic that may be extended to all Type II and Type III arteries.
Type II arteries are prone to spasm because of the higher contractility of splanchnic arteries, which is also responsible for the tremendous changes to splanchnic artery blood flow under various circumstances to accommodate the function of the alimentary tract. The flow increases after meals and decreases under critical situations. In contrast, Type I arterial grafts (somatic arteries) are less reactive than Type II grafts because they are mainly “less reactive” conduit arteries except at the end of the artery, which functions as a muscular regulator for blood flow, as demonstrated in the human IMA (64-66).
Type III arteries are located in RA of the limbs and have a higher tendency for spasm compared to somatic arteries (Type I). It is a common clinical observation that the extremities of arteries are prone to spasm in either physiological conditions or under pathological conditions, as seen in Raynaud’s disease.
The prevalence of vasospasm in arterial grafts also correlates with their endothelial function. The Type I artery, particularly the IMA, releases more NO and has higher EDHF-mediated relaxation and hyperpolarization than the Type III artery RA (37,38) and may prove to have the best endothelial function among arterial grafts. This certainly contributes to the superior patency of this graft. Importantly, most of the studies comparing the endothelial function of arterial grafts are performed in vascular segments taken from coronary artery bypass grafting patients who are old and have coronary disease; the superior endothelial function of the IMA may merely reflect the fact that this artery is usually free from atherosclerosis whereas other type (Type II and III) arteries are usually more involved with atherosclerotic changes that diminish the endothelial function.
Because Type II and III arteries are prone to spasm due to higher contractility, they require more active pharmacological intervention (34,37,48).
There are clinical implications relating to this classification. Firstly, this clinical classification may be useful when searching for new arterial grafts or predicting the behavior of a graft. Type I arteries may be less spastic than Type II and Type III which posits their advantageous use perioperatively, particularly when their most reactive portion, the distal section, is trimmed off (64,65,115). In fact, in common clinical practice since the 1980s, the IMA has been used as the first choice of arterial grafts and is always used to graft the most important coronary artery, the LAD.
With this classification system, the surgeon is able to predict the behavior of the arterial graft and choose an optimal pharmacological method to overcome vasospasm. For the Type II or III arteries, more active pharmacological intervention is necessary in order to prevent or treat vasospasm in these arteries.
The most important issue in coronary grafting for anastomosis is the long-term patency of the graft, which is dependent on technical factors and endothelial function. Vasospasm is also related to the long-term patency as seen in the early use of the RA, which encountered a severe problem with spasms reducing the patency of the RA graft (40,41). Even in the largest series from Tatoulis and colleagues who are highly experienced with arterial grafting, the patency for RA was 89% at 4 years, compared to 98% the patency for the left IMA at 5 years (19). Although technical factors, such as target vessels, may be involved in this difference, the aforementioned differences in the endothelial function regarding NO and EDHF between the IMA and RA may play a role.
Furthermore, arteries of the same type may have different long-term patency due to the presence of atherosclerosis either in the native artery, the graft, or other factors. While the Type I artery IMA has been well established with its superior long-term patency, the IEA has lower patency than IMA (116,117). Several factors may account for this as: (I) the IEA is used as a free graft; (II) the IEA is very small at the distal end which increases technical difficulties (118); (III) the size of the proximal IEA is less than 2 mm which causes difficulty for the aortic anastomosis (118); and (IV) the IEA, at least in the proximal part, has a higher incidence of atherosclerosis which may influence patency (4). Therefore, the proposed new technique involves trimming off the very small section of the distal end of the IEA and the atherosclerotic proximal end so it can be used as a part of a composite graft (118). In this way, use of the IEA may reach a similar patency rate comparable to the IMA.
The patency rate of Type II and III arteries not well established compared to the IMA. Suma reported that the cumulative patency rate estimated by the Kaplan-Meier method was 96.6% at 1 month, 91.4% at 1 year, 80.5% at 5 years, and 62.5% at 10 years (119). Causes of late occlusion were primary anastomotic stenosis and anastomosis to a less critically stenosed coronary artery. Voutilainers and associates (120) reported that 82.1% (23/26) of GEA grafts were patent at 5 years. From those studies, the patency of the GEA, as a Type II artery, is acceptable but inferior to that of the IMA which was 95% at 10 years and 88% at 15 years (19).
The patency rate of the RA was poorer, with 35% incidence of narrowing or occlusion of the RA (121). With modified technique, avoiding skeletonization and using calcium antagonists, the early patency increased to 93.5% at 9 months (41), 83% at 5 years (122) in Acar’s group and 93.1% to 95.7% in other groups (123,124) at 3-21 months in the early period of the use of RA. In addition, Tatoulis and associates reported that the RA patency at 1 year was 96% and at 4 years it was 89% (19).
These results suggest that Type II and III arteries may have inferior patency to that of the IMA. However, if vasospasm and technical problems can be overcome, and endothelial function is well preserved, then the patency of Type II and III arteries may significantly improve.
In summary, arterial grafts are biologically divergent conductance arteries which can be functionally and clinically classified as three types. Type II (such as GEA) and Type III (such as RA) are more readily spastic than Type I (such as IMA). In order to obtain the best results, antispasm therapy, preservation of endothelial function, and other technical modifications are essential particularly in Type II & III arteries.
Pharmacological management: antispasm protocols for conduits
Pharmacological management in CABG is a complex topic which includes two major aspects. Firstly, systematic administration of pharmacological agents is used in order to maintain cardiac output and graft function during the pre-, intra-, and post-operative period, and secondly, arterial conduits are pharmacologically managed with both systematic and topical methods.
There are a few antispasm protocols available in different units around the world. We present the following protocols used in our practice, which are also used by other cardiac surgeons in various countries.
The GW HE Protocol (modified UHK Protocol) for arterial grafting
Based on the above pharmacological studies, we have developed an antispasm protocol, the GW HE Protocol (modified UHK Protocol) for use in arterial grafting (77,78). While the solution (VG solution) was originally developed for IMA and saphenous vein harvesting and was later expanded to include RA grafting, it should be also useful for arterial grafting using other grafts. The use of VG solution in RA grafting necessitates the inclusion of pre-, intra-, and post-operative management. The necessity of postoperative calcium antagonists for IMA grafting, however, should be decided by the surgeon and cardiologist according to the patient’s condition.
Preoperative for RA grafting (not necessary for using other grafts)
- Allen Test for both arms;
- Doppler flow examination for the ulnar artery during Allen test and for the RA flow to demonstrate its patency.
Intraoperative
- Use verapamil plus nitroglycerin (VG) solution, which has a concentration of approximately 30 µmol/L of verapamil and NTG in an isotonic solution of pH 7.4. The components include (75-78):
- Verapamil hydrochloride, 5 mg;
- Nitroglycerin (NTG), 2.5 mg;
- Heparin, 500 unit;
- 8.4% NaHCO3, 0.2 mL;
- Ringer’s solution, 300 mL.
- The RA is removed immediately after dissection from the arm and stored in the VG solution at the room temperature;
- Once the harvesting of the RA has initiated, low dose nicardipine (0.5 mg per hour; 5 mg in 100 mL D5W, IV at 10 mL per hour) is given systematically.
Postoperative
-
Nicardipine is infused IV at the same dose until the patient is able to take oral calcium antagonists;
-
Continuing a low dose of one of the calcium antagonists for at least 6-12 months. This can be nicardipine 20 mg twice a day; or verapamil 120-240 mg per day (A test dose 120 mg is recommended), or diltiazem at an appropriate low dose. The choice of the calcium antagonist (nicardipine/diltiazem/verapamil) is based on availability, the patient’s condition, particularly the heart rate, as well as the preference of the cardiologist. Use of beta-blockers should be cautious when some of the calcium antagonists are given.
During harvesting of the IMA, this solution is sprayed on the pedicle as well as injected into the lumen. The solution was also shown to be effective in intraluminal injection after CABG to reverse spasm of grafts and coronary arteries (110).
The GW HE antispasm solution No. 2. (NG solution) for arterial grafting
Design of the nicardipine plus nitroglycerin (NG) cocktail
Alongside its limited availability, verapamil also has a bradycardiac effect which may prevent the simultaneous use of β-blocker. Additionally, the advent and development of new generations of calcium antagonists have led to decreased use of verapamil. For these reasons, we designed a new cocktail that is composed of a second generation of the dihydropyridine calcium antagonist, nicardipine and nitroglycerin. Following testing, effectiveness of this cocktail was demonstrated on both human IMA and RA (125).
We tested the effect of nicardipine and NTG at the concentration of 30 µMol/L (–4.5 logM) on the human IMA and RA segments in the organ chamber. From previous studies (42,53,71,74-78,81,84,91-93,96,110), this concentration was expected to have maximal or nearly maximal effect.
This cocktail consists of 30 µMol/L (–4.5 logM) nicardipine and 60 µMol/L (–4.3 logM) NTG in an isotonic solution of pH 7.1. The components of the clinical (NG) cocktail include:
- Nicardipine hydrochloride, 5 mg;
- NTG, 5 mg;
- 8.4% NaHCO3, 0.3 mL*;
- Normosol-R solution, 300 mL;
- (heparin, 500 Unit could be added).
*The pH of NG (nicardipine hydrochloride 5 mg and NTG 5 mg) in Normosol-R solution (300 mL) without adding NaHCO3 is 6.6. When a volume greater than 0.3 mL of 8.4% NaHCO3 is added, the solution becomes gradually turbid at pH of 7.1-7.4. This however, does not affect the antispastic effect (125). However, if nicardipine hydrochloride (5 mg) and NTG (5 mg) are added to Multiple Electrolytes Injection (Baxter International Inc, Shanghai, China) with pH of 7.4, the cocktail solution is clear and there is no need to add NaHCO3 (126).
In summary, we have presented two solutions for use as part of an antispasm protocol during arterial grafting. Our experimental studies (75-78,125) have demonstrated that these solutions are effective vasodilator cocktails that relax vasoconstriction caused by all mechanisms, including depolarizing and receptor mechanisms. This is superior compared to other solutions such as α-adrenoceptor antagonists which are only effective at reversing adrenoceptor-mediated spasm, and calcium antagonists alone which are only effective at reversing depolarizing agent (K+)-mediated spasm. Our clinical trials have demonstrated that these are excellent vasodilators both to reverse or prevent spasm (75,76,110,126). Most recently, we have also demonstrated that the use of antispasm nicardipine and nitroglycerin cocktail solution increases IMA graft flow during off-pump coronary artery bypass grafting (126).
Acknowledgements
Disclosure: The author declares no conflict of interest.
References
- Loop FD, Lytle BW, Cosgrove DM, et al. Influence of the internal-mammary-artery graft on 10-year survival and other cardiac events. N Engl J Med 1986;314:1-6. [PubMed]
- Barner HB, Standeven JW, Reese J. Twelve-year experience with internal mammary artery for coronary artery bypass. J Thorac Cardiovasc Surg 1985;90:668-75. [PubMed]
- He GW, Angus JA, Rosenfeldt FL. Reactivity of the canine isolated internal mammary artery, saphenous vein, and coronary artery to constrictor and dilator substances: relevance to coronary bypass graft surgery. J Cardiovasc Pharmacol 1988;12:12-22. [PubMed]
- van Son JA, Smedts F, Vincent JG, et al. Comparative anatomic studies of various arterial conduits for myocardial revascularization. J Thorac Cardiovasc Surg 1990;99:703-7. [PubMed]
- Lüscher TF, Diederich D, Siebenmann R, et al. Difference between endothelium-dependent relaxation in arterial and in venous coronary bypass grafts. N Engl J Med 1988;319:462-7. [PubMed]
- Liu ZG, Ge ZD, He GW. Difference in endothelium-derived hyperpolarizing factor-mediated hyperpolarization and nitric oxide release between human internal mammary artery and saphenous vein. Circulation 2000;102:III296-301. [PubMed]
- Zhang RZ, Yang Q, Yim AP, et al. Different role of nitric oxide and endothelium-derived hyperpolarizing factor in endothelium-dependent hyperpolarization and relaxation in porcine coronary arterial and venous system. J Cardiovasc Pharmacol 2004;43:839-50. [PubMed]
- Carpentier A, Guermonprez JL, Deloche A, et al. The aorta-to-coronary radial artery bypass graft. A technique avoiding pathological changes in grafts. Ann Thorac Surg 1973;16:111-21. [PubMed]
- Pym J, Brown PM, Charrette EJ, et al. Gastroepiploic-coronary anastomosis. A viable alternative bypass graft. J Thorac Cardiovasc Surg 1987;94:256-9. [PubMed]
- Puig LB, Ciongolli W, Cividanes GV, et al. Inferior epigastric artery as a free graft for myocardial revascularization. J Thorac Cardiovasc Surg 1990;99:251-5. [PubMed]
- Buche M, Schoevaerdts JC, Louagie Y, et al. Use of the inferior epigastric artery for coronary bypass. J Thorac Cardiovasc Surg 1992;103:665-70. [PubMed]
- Edwards WS, Lewis CE, Blakeley WR, et al. Coronary artery bypass with internal mammary and splenic artery grafts. Ann Thorac Surg 1973;15:35-40. [PubMed]
- Mills NL, Dupin CL, Everson CT, et al. The subscapular artery: an alternative conduit for coronary bypass. J Card Surg 1993;8:66-71. [PubMed]
- Shatapathy P, Aggarwal BK, Punnen J. Inferior mesenteric artery as a free arterial conduit for myocardial revascularization. J Thorac Cardiovasc Surg 1997;113:210-1. [PubMed]
- Tatsumi TO, Tanaka Y, Kondoh K, et al. Descending branch of lateral femoral circumflex artery as a free graft for myocardial revascularization: a case report. J Thorac Cardiovasc Surg 1996;112:546-7. [PubMed]
- Buxton BF, Chan AT, Dixit AS, et al. Ulnar artery as a coronary bypass graft. Ann Thorac Surg 1998;65:1020-4. [PubMed]
- van Son JA, Smedts F, Korving J, et al. Intercostal artery: histomorphometric study to assess its suitability as a coronary bypass graft. Ann Thorac Surg 1993;56:1078-81. [PubMed]
- Tatoulis J, Buxton BF, Fuller JA, et al. Long-term patency of 1108 radial arterial-coronary angiograms over 10 years. Ann Thorac Surg 2009;88:23-9; discussion 29-30. [PubMed]
- Tatoulis J, Buxton BF, Fuller JA. Patencies of 2127 arterial to coronary conduits over 15 years. Ann Thorac Surg 2004;77:93-101. [PubMed]
- Schwann TA, Zacharias A, Riordan CJ, et al. Sequential radial artery grafts for multivessel coronary artery bypass graft surgery: 10-year survival and angiography results. Ann Thorac Surg 2009;88:31-9. [PubMed]
- Hayward PA, Hare DL, Gordon I, et al. Effect of radial artery or saphenous vein conduit for the second graft on 6-year clinical outcome after coronary artery bypass grafting. Results of a randomised trial. Eur J Cardiothorac Surg 2008;34:113-7. [PubMed]
- Schwann TA, Zacharias A, Riordan CJ, et al. Does radial use as a second arterial conduit for coronary artery bypass grafting improve long-term outcomes in diabetics? Eur J Cardiothorac Surg 2008;33:914-23. [PubMed]
- Nezić DG, Knezević AM, Milojević PS, et al. The fate of the radial artery conduit in coronary artery bypass grafting surgery. Eur J Cardiothorac Surg 2006;30:341-6. [PubMed]
- Eda T, Matsuura A, Miyahara K, et al. Transplantation of the free gastroepiploic artery graft for myocardial revascularization: long-term clinical and angiographic results. Ann Thorac Surg 2008;85:880-4. [PubMed]
- Hirose H, Amano A, Takanashi S, et al. Coronary artery bypass grafting using the gastroepiploic artery in 1,000 patients. Ann Thorac Surg 2002;73:1371-9. [PubMed]
- Suma H, Wanibuchi Y, Furuta S, et al. Comparative study between the gastroepiploic and the internal thoracic artery as a coronary bypass graft. Size, flow, patency, histology. Eur J Cardiothorac Surg 1991;5:244-7. [PubMed]
- Suma H, Tanabe H, Yamada J, et al. Midterm results for use of the skeletonized gastroepiploic artery graft in coronary artery bypass. Circ J 2007;71:1503-5. [PubMed]
- Lytle BW, Cosgrove DM, Ratliff NB, et al. Coronary artery bypass grafting with the right gastroepiploic artery. J Thorac Cardiovasc Surg 1989;97:826-31. [PubMed]
- Suma H, Wanibuchi Y, Terada Y, et al. The right gastroepiploic artery graft. Clinical and angiographic midterm results in 200 patients. J Thorac Cardiovasc Surg 1993;105:615-22; discussion 623. [PubMed]
- Grandjean JG, Boonstra PW, den Heyer P, et al. Arterial revascularization with the right gastroepiploic artery and internal mammary arteries in 300 patients. J Thorac Cardiovasc Surg 1994;107:1309-15; discussion 1315-6. [PubMed]
- van Son JA, Smedts FM, Yang CQ, et al. Morphometric study of the right gastroepiploic and inferior epigastric arteries. Ann Thorac Surg 1997;63:709-15. [PubMed]
- Dignan RJ, Yeh T Jr, Dyke CM, et al. Reactivity of gastroepiploic and internal mammary arteries. Relevance to coronary artery bypass grafting. J Thorac Cardiovasc Surg 1992;103:116-22; discussion 122-3. [PubMed]
- Chardigny C, Jebara VA, Acar C, et al. Vasoreactivity of the radial artery. Comparison with the internal mammary and gastroepiploic arteries with implications for coronary artery surgery. Circulation 1993;88:II115-27. [PubMed]
- He GW, Yang CQ. Comparison among arterial grafts and coronary artery. An attempt at functional classification. J Thorac Cardiovasc Surg 1995;109:707-15. [PubMed]
- He GW, Yang CQ, Starr A. Overview of the nature of vasoconstriction in arterial grafts for coronary operations. Ann Thorac Surg 1995;59:676-83. [PubMed]
- He GW, Acuff TE, Ryan WH, et al. Functional comparison between the human inferior epigastric artery and internal mammary artery. Similarities and differences. J Thorac Cardiovasc Surg 1995;109:13-20. [PubMed]
- He GW, Liu ZG. Comparison of nitric oxide release and endothelium-derived hyperpolarizing factor-mediated hyperpolarization between human radial and internal mammary arteries. Circulation 2001;104:I344-9. [PubMed]
- He GW, Fan L, Grove KL, et al. Expression and function of endothelial nitric oxide synthase messenger RNA and protein are higher in internal mammary than in radial arteries. Ann Thorac Surg 2011;92:845-50. [PubMed]
- Suma H. Spasm of the gastroepiploic artery graft. Ann Thorac Surg 1990;49:168-9. [PubMed]
- Fisk RL, Brooks CH, Callaghan JC, et al. Experience with the radial artery graft for coronary artery bypass. Ann Thorac Surg 1976;21:513-8. [PubMed]
- Acar C, Jebara VA, Portoghese M, et al. Revival of the radial artery for coronary artery bypass grafting. Ann Thorac Surg 1992;54:652-9; discussion 659-60. [PubMed]
- He GW, Rosenfeldt FL, Buxton BF, et al. Reactivity of human isolated internal mammary artery to constrictor and dilator agents. Implications for treatment of internal mammary artery spasm. Circulation 1989;80:I141-50. [PubMed]
- Liu MH, Floten HS, Furnary AP, et al. Inhibition of vasoconstriction by angiotensin receptor antagonist GR117289C in arterial grafts. Ann Thorac Surg 2000;70:2064-9. [PubMed]
- Wei W, Floten HS, He GW. Interaction between vasodilators and vasopressin in internal mammary artery and clinical significance. Ann Thorac Surg 2002;73:516-22. [PubMed]
- Luu TN, Dashwood MR, Chester AH, et al. Action of vasoactive intestinal peptide and distribution of its binding sites in vessels used for coronary artery bypass grafts. Am J Cardiol 1993;71:1278-82. [PubMed]
- Chen ZW, Yang Q, Huang Y, et al. Human urotensin II in internal mammary and radial arteries of patients undergoing coronary surgery. Vascul Pharmacol 2010;52:70-6. [PubMed]
- Bai XY, Liu XC, Yang Q, et al. The interaction between human urotensin II and vasodilator agents in human internal mammary artery with possible clinical implications. Ann Thorac Surg 2011;92:610-6. [PubMed]
- He GW, Yang CQ. Radial artery has higher receptor-mediated contractility but similar endothelial function compared with mammary artery. Ann Thorac Surg 1997;63:1346-52. [PubMed]
- He GW, Shaw J, Hughes CF, et al. Predominant alpha 1-adrenoceptor-mediated contraction in the human internal mammary artery. J Cardiovasc Pharmacol 1993;21:256-63. [PubMed]
- He GW, Yang CQ. Characteristics of adrenoceptors in the human radial artery: clinical implications. J Thorac Cardiovasc Surg 1998;115:1136-41. [PubMed]
- Seo B, Oemar BS, Siebenmann R, et al. Both ETA and ETB receptors mediate contraction to endothelin-1 in human blood vessels. Circulation 1994;89:1203-8. [PubMed]
- Yildiz O, Ciçek S, Ay I, et al. 5-HT1-like receptor-mediated contraction in the human internal mammary artery. J Cardiovasc Pharmacol 1996;28:6-10. [PubMed]
- He GW, Yang CQ. Comparison of nitroprusside and nitroglycerin in inhibition of angiotensin II and other vasoconstrictor-mediated contraction in human coronary bypass conduits. Br J Clin Pharmacol 1997;44:361-7. [PubMed]
- He GW, Yang CQ. Effect of thromboxane A2 antagonist GR32191B on prostanoid and nonprostanoid receptors in the human internal mammary artery. J Cardiovasc Pharmacol 1995;26:13-9. [PubMed]
- Liu JJ, Phillips PA, Burrell LM, et al. Human internal mammary artery responses to non-peptide vasopressin antagonists. Clin Exp Pharmacol Physiol 1994;21:121-4. [PubMed]
- Ochiai M, Ohno M, Taguchi J, et al. Responses of human gastroepiploic arteries to vasoactive substances: comparison with responses of internal mammary arteries and saphenous veins. J Thorac Cardiovasc Surg 1992;104:453-8. [PubMed]
- Mügge A, Barton MR, Cremer J, et al. Different vascular reactivity of human internal mammary and inferior epigastric arteries in vitro. Ann Thorac Surg 1993;56:1085-9. [PubMed]
- He GW, Buxton B, Rosenfeldt FL, et al. Weak beta-adrenoceptor-mediated relaxation in the human internal mammary artery. J Thorac Cardiovasc Surg 1989;97:259-66. [PubMed]
- Wei W, Jin H, Chen ZW, et al. Vascular endothelial growth factor-induced nitric oxide- and PGI2-dependent relaxation in human internal mammary arteries: a comparative study with KDR and Flt-1 selective mutants. J Cardiovasc Pharmacol 2004;44:615-21. [PubMed]
- Chen ZW, Huang Y, Yang Q, et al. Urocortin-induced relaxation in the human internal mammary artery. Cardiovasc Res 2005;65:913-20. [PubMed]
- He GW, Yang CQ, Graier WF, et al. Hyperkalemia alters EDHF-mediated hyperpolarization and relaxation in coronary arteries. Am J Physiol 1996;271:H760-7. [PubMed]
- Ge ZD, Zhang XH, Fung PC, et al. Endothelium-dependent hyperpolarization and relaxation resistance to N(G)-nitro-L-arginine and indomethacin in coronary circulation. Cardiovasc Res 2000;46:547-56. [PubMed]
- Williams PL, Warwick R, Dyson M, et al. eds. Gray’s anatomy. New York: Churchill livingstone, 1989:213-9.
- He GW. Contractility of the human internal mammary artery at the distal section increases toward the end. Emphasis on not using the end of the internal mammary artery for grafting. J Thorac Cardiovasc Surg 1993;106:406-11. [PubMed]
- He GW, Acuff TE, Yang CQ, et al. Middle and proximal sections of the human internal mammary artery are not “passive conduits”. J Thorac Cardiovasc Surg 1994;108:741-6. [PubMed]
- He GW, Yang CQ. Comparison among arterial grafts and coronary artery. An attempt at functional classification. J Thorac Cardiovasc Surg 1995;109:707-15. [PubMed]
- Sims FH. A comparison of coronary and internal mammary arteries and implications of the results in the etiology of arteriosclerosis. Am Heart J 1983;105:560-6. [PubMed]
- Suma H. Gastroepiploic artery graft: coronary artery bypass graft in patients with diseased ascending aorta - using an aortic no-touch technique. Operative techniques in Cardiac & Thoracic Surgery 1996;1:185-95.
- Barner HB, Barnett MG. Fifteen- to twenty-one-year angiographic assessment of internal thoracic artery as a bypass conduit. Ann Thorac Surg 1994;57:1526-8. [PubMed]
- Desai ND, Cohen EA, Naylor CD, et al. A randomized comparison of radial-artery and saphenous-vein coronary bypass grafts. N Engl J Med 2004;351:2302-9. [PubMed]
- Cooper GJ, Locke TJ. The in vitro response of human internal mammary artery to vasodilators. J Thorac Cardiovasc Surg 1994;107:1155-7. [PubMed]
- He GW, Yang CQ. Pharmacological studies and guidelines for the use of vasodilators for arterial grafts. In: He GW. eds. Arterial grafting for coronary artery bypass surgery, 2nd Ed. Berlin: Springer, 2006:39-44.
- Jett GK, Guyton RA, Hatcher CR Jr, et al. Inhibition of human internal mammary artery contractions. An in vitro study of vasodilators. J Thorac Cardiovasc Surg 1992;104:977-82. [PubMed]
- He GW, Yang CQ, Mack MJ, et al. Interaction between endothelin and vasodilators in the human internal mammary artery. Br J Clin Pharmacol 1994;38:505-12. [PubMed]
- He GW, Rosenfeldt FL, Angus JA. Pharmacological relaxation of the saphenous vein during harvesting for coronary artery bypass grafting. Ann Thorac Surg 1993;55:1210-7. [PubMed]
- He GW, Buxton BF, Rosenfeldt FL, et al. Pharmacologic dilatation of the internal mammary artery during coronary bypass grafting. J Thorac Cardiovasc Surg 1994;107:1440-4. [PubMed]
- He GW, Yang CQ. Use of verapamil and nitroglycerin solution in preparation of radial artery for coronary grafting. Ann Thorac Surg 1996;61:610-4. [PubMed]
- He GW. Verapamil plus nitroglycerin solution maximally preserves endothelial function of the radial artery: comparison with papaverine solution. J Thorac Cardiovasc Surg 1998;115:1321-7. [PubMed]
- Cunningham JN Jr. Papaverine hydrochloride preservation of vein grafts. J Thorac Cardiovasc Surg 1982;84:933. [PubMed]
- Chavanon O, Cracowski JL, Hacini R, et al. Effect of topical vasodilators on gastroepiploic artery graft. Ann Thorac Surg 1999;67:1295-8. [PubMed]
- He GW, Shaw J, Yang CQ, et al. Inhibitory effects of glyceryl trinitrate on alpha-adrenoceptor mediated contraction in the human internal mammary artery. Br J Clin Pharmacol 1992;34:236-43. [PubMed]
- Cooper GJ, Wilkinson GA, Angelini GD. Overcoming perioperative spasm of the internal mammary artery: which is the best vasodilator? J Thorac Cardiovasc Surg 1992;104:465-8. [PubMed]
- He GW, Yang CO, Gately H, et al. Potential greater than additive vasorelaxant actions of milrinone and nitroglycerin on human conduit arteries. Br J Clin Pharmacol 1996;41:101-7. [PubMed]
- He GW, Yang CQ. Comparison of the vasorelaxant effect of nitroprusside and nitroglycerin in the human radial artery in vitro. Br J Clin Pharmacol 1999;48:99-104. [PubMed]
- Zabeeda D, Medalion B, Jackobshvilli S, et al. Comparison of systemic vasodilators: effects on flow in internal mammary and radial arteries. Ann Thorac Surg 2001;71:138-41. [PubMed]
- Shapira OM, Xu A, Vita JA, et al. Nitroglycerin is superior to diltiazem as a coronary bypass conduit vasodilator. J Thorac Cardiovasc Surg 1999;117:906-11. [PubMed]
- Cable DG, Caccitolo JA, Pearson PJ, et al. New approaches to prevention and treatment of radial artery graft vasospasm. Circulation 1998;98:II15-21; discussion II21-2.
- Lobato EB, Janelle GM, Urdaneta F, et al. Comparison of milrinone versus nitroglycerin, alone and in combination, on grafted internal mammary artery flow after cardiopulmonary bypass: effects of alpha-adrenergic stimulation. J Cardiothorac Vasc Anesth 2001;15:723-7. [PubMed]
- He GW, Acuff TE, Ryan WH, et al. Inhibitory effects of calcium antagonists on alpha-adrenoceptor-mediated contraction in the human internal mammary artery. Br J Clin Pharmacol 1994;37:173-9. [PubMed]
- Rosenfeldt FL, He GW, Buxton BF, et al. Pharmacology of coronary artery bypass grafts. Ann Thorac Surg 1999;67:878-88. [PubMed]
- He GW, Yang CQ. Comparative study on calcium channel antagonists in the human radial artery: clinical implications. J Thorac Cardiovasc Surg 2000;119:94-100. [PubMed]
- Wei W, Floten HS, He GW. Interaction between vasodilators and vasopressin in internal mammary artery and clinical significance. Ann Thorac Surg 2002;73:516-22. [PubMed]
- He GW, Yang CQ. Inhibition of vasoconstriction by phosphodiesterase III inhibitor milrinone in human conduit arteries used as coronary bypass grafts. J Cardiovasc Pharmacol 1996;28:208-14. [PubMed]
- Liu JJ, Doolan LA, Xie B, et al. Direct vasodilator effect of milrinone, an inotropic drug, on arterial coronary bypass grafts. FANZCA. J Thorac Cardiovasc Surg 1997;113:108-13. [PubMed]
- He GW. Effect of milrinone on coronary artery bypass grafts. J Thorac Cardiovasc Surg 1997;114:302-4. [PubMed]
- He GW, Yang CQ. Vasorelaxant effect of phosphodiesterase-inhibitor milrinone in the human radial artery used as coronary bypass graft. J Thorac Cardiovasc Surg 2000;119:1039-45. [PubMed]
- Wei W, Yang CQ, Furnary A, et al. Greater vasopressin-induced vasoconstriction and inferior effects of nitrovasodilators and milrinone in the radial artery than in the internal thoracic artery. J Thorac Cardiovasc Surg 2005;129:33-40. [PubMed]
- Cracowski JL, Stanke-Labesque F, Chavanon O, et al. Vasorelaxant actions of enoximone, dobutamine, and the combination on human arterial coronary bypass grafts. J Cardiovasc Pharmacol 1999;34:741-8. [PubMed]
- He GW, Yang CQ. Inhibition of vasoconstriction by the thromboxane A2 antagonist GR32191B in the human radial artery. Br J Clin Pharmacol 1999;48:207-15. [PubMed]
- He GW, Yang CQ. Inhibition of vasoconstriction by potassium channel opener aprikalim in human conduit arteries used as bypass grafts. Br J Clin Pharmacol 1997;44:353-9. [PubMed]
- Liu MH, Floten HS, Furnary AP, et al. Effects of potassium channel opener aprikalim on the receptor-mediated vasoconstriction in the human internal mammary artery. Ann Thorac Surg 2001;71:636-41. [PubMed]
- Ren Z, Floten S, Furnary A, et al. Effects of potassium channel opener KRN4884 on human conduit arteries used as coronary bypass grafts. Br J Clin Pharmacol 2000;50:154-60. [PubMed]
- Mussa S, Guzik TJ, Black E, et al. Comparative efficacies and durations of action of phenoxybenzamine, verapamil/nitroglycerin solution, and papaverine as topical antispasmodics for radial artery coronary bypass grafting. J Thorac Cardiovasc Surg 2003;126:1798-805. [PubMed]
- Corvera JS, Morris CD, Budde JM, et al. Pretreatment with phenoxybenzamine attenuates the radial artery’s vasoconstrictor response to alpha-adrenergic stimuli. J Thorac Cardiovasc Surg 2003;126:1549-54. [PubMed]
- Conant AR, Shackcloth MJ, Oo AY, et al. Phenoxybenzamine treatment is insufficient to prevent spasm in the radial artery: the effect of other vasodilators. J Thorac Cardiovasc Surg 2003;126:448-54. [PubMed]
- Liu MH, Floten SH, Yang Q, et al. Inhibition of vasoconstriction by AJ-2615, a novel calcium antagonist with alpha(1)-adrenergic receptor blocking activity in human conduit arteries used as bypass grafts. Br J Clin Pharmacol 2001;52:279-87. [PubMed]
- Liu MH, Floten HS, Furnary AP, et al. Inhibition of vasoconstriction by angiotensin receptor antagonist GR117289C in arterial grafts. Ann Thorac Surg 2000;70:2064-9. [PubMed]
- Liu JJ, Johnston CI, Buxton BF. Synergistic effect of nisoldipine and nitroglycerin on human internal mammary artery. J Pharmacol Exp Ther 1994;268:434-40. [PubMed]
- Chanda J, Brichkov I, Canver CC. Prevention of radial artery graft vasospasm after coronary bypass. Ann Thorac Surg 2000;70:2070-4. [PubMed]
- He GW, Fan KY, Chiu SW, et al. Injection of vasodilators into arterial grafts through cardiac catheter to relieve spasm. Ann Thorac Surg 2000;69:625-8. [PubMed]
- Kiemeneij F, Vajifdar BU, Eccleshall SC, et al. Evaluation of a spasmolytic cocktail to prevent radial artery spasm during coronary procedures. Catheter Cardiovasc Interv 2003;58:281-4. [PubMed]
- Koike R, Suma H, Kondo K, et al. Pharmacological response of internal mammary artery and gastroepiploic artery. Ann Thorac Surg 1990;50:384-6. [PubMed]
- Liu ZG, Liu XC, Yim AP, et al. Direct measurement of nitric oxide release from saphenous vein: abolishment by surgical preparation. Ann Thorac Surg 2001;71:133-7. [PubMed]
- Arterial grafts for coronary artery bypass grafting: biological characteristics, functional classification, and clinical choice. Ann Thorac Surg 1999;67:277-84. [PubMed]
- He GW. Spasm of internal mammary artery: is it a secret? J Thorac Cardiovasc Surg 1993;106:381-2. [PubMed]
- Cremer J, Mügge A, Schulze M, et al. The inferior epigastric artery for coronary bypass grafting. Functional assessment and clinical results. Eur J Cardiothorac Surg 1993;7:423-7. [PubMed]
- Perrault LP, Carrier M, Hebert Y, et al. Early experience with the inferior epigastric artery in coronary artery bypass grafting. A word of caution. J Thorac Cardiovasc Surg 1993;106:928-30. [PubMed]
- Calafiore AM. Use of the inferior epigastric artery for coronary revascularization. Operatice techniques in Cardiac & Thoracic Surgery 1996;1:147-59.
- Suma H, Isomura T, Horii T, et al. Late angiographic result of using the right gastroepiploic artery as a graft. J Thorac Cardiovasc Surg 2000;120:496-8. [PubMed]
- Voutilainen S, Verkkala K, Järvinen A, et al. Angiographic 5-year follow-up study of right gastroepiploic artery grafts. Ann Thorac Surg 1996;62:501-5. [PubMed]
- Geha AS, Krone RJ, McCormick JR, et al. Selection of coronary bypass. Anatomic, physiological, and angiographic considerations of vein and mammary artery grafts. J Thorac Cardiovasc Surg 1975;70:414-31. [PubMed]
- Acar C, Ramsheyi A, Pagny JY, et al. The radial artery for coronary artery bypass grafting: clinical and angiographic results at five years. J Thorac Cardiovasc Surg 1998;116:981-9. [PubMed]
- Calafiore AM, Di Giammarco G, Teodori G, et al. Radial artery and inferior epigastric artery in composite grafts: improved midterm angiographic results. Ann Thorac Surg 1995;60:517-23; discussion 523-4. [PubMed]
- Brodman RF, Frame R, Camacho M, et al. Routine use of unilateral and bilateral radial arteries for coronary artery bypass graft surgery. J Am Coll Cardiol 1996;28:959-63. [PubMed]
- He GW, Fan L, Furnary A, et al. A new antispastic solution for arterial grafting: nicardipine and nitroglycerin cocktail in preparation of internal thoracic and radial arteries for coronary surgery. J Thorac Cardiovasc Surg 2008;136:673-80, 680.e1-2.
- Zheng SY, Wu M, Huang JS, et al. Use of antispastic nicardipine and nitroglycerin (NG) cocktail solution increases graft flow during off-pump coronary artery bypass grafting. J Cardiovasc Surg (Torino) 2012;53:783-8. [PubMed]