How to avoid transcatheter aortic valve replacement explant as the second valve procedure: image assessment for the index transcatheter aortic valve replacement
Introduction
The current guidelines for managing valvular heart disease changed the treatment focus for aortic stenosis (AS) to shared decision-making with the patient regarding recovery goals and the potential need for valve reoperation, shifting from historical metrics of determining risk stratification and feasibility (1,2). In the United States (US), symptomatic AS patients over 65 years old with at least 20 years of life expectancy have the choice of transcatheter aortic valve replacement (TAVR) or surgical aortic valve replacement (SAVR) (1). European Guidelines employ an age cutoff of 75 years, similar to patients in the low-risk randomized controlled trials and the median age of low-risk patients in the Society of Thoracic Surgery (STS) national database (3-6). Databases of US patients consistently show that the fastest growing demographic receiving TAVR are patients under 65 years old, with 47.5% in the Vizient Clinical Database (7), 45.7% in the California registry (60 years old or less) (8), and 51.7% in the Northern New England Cardiovascular Group (9). Although age alone does not necessarily determine procedural risk or life expectancy (10,11), many who receive biological valves will require a second valve in their lifetime. A patient’s desire for a less invasive operation and faster recovery must be balanced with the long-term objectives of the lifetime management of valvular heart disease.
While we acknowledge that surgical mechanical valves and the Ross procedure offer the greatest opportunity for only one aortic valve replacement (AVR) in a patient’s lifetime, the reality is that most patients do not want a lifetime of Coumadin, and the Ross procedure is reserved for very young patients who make up only a small portion of patients with severe AS (1,12). A biological valve relieves the mechanical obstruction of AS but does not cure the valve disease. Instead, SAVR or TAVR with a bioprosthesis trades native AS for a biologic valve with a finite, unpredictable lifespan that will eventually fail if the patient lives long enough.
Although there are many considerations when discussing the “best” first valve for a specific patient, such as age, anticoagulation tolerance, durability of available valves, presence of bicuspid aortic valve or concomitant aortopathy, existing comorbidities, aortic root anatomy, additional valve or coronary disease, and coronary re-access, the feasibility of a second valve must be considered at the pre-planning stage of the first valve. Heart teams must attempt to select a first valve that will provide a high likelihood of lifetime durability and be a platform for a second valve if the first valve fails. Moreover, with limited long-term durability for transcatheter aortic valves (TAVs) and only 5 years of follow-up in the US low-risk AS trials, planning for one valve in a patient’s lifetime is challenging. Herein, we review our strategy around selecting an initial valve to prevent the need for a second valve or plan appropriately if the need arises.
Valve durability
Historically, biological valve durability was based on small single-armed observational series (13) typically sponsored by the valve manufacturers. Surgical valves were assumed to have 10–15-year durability, with only medical therapy as an alternative. This durability was defined as freedom from valve reintervention and failed to account for patients not offered a second operation or who died with a failed valve. Nonetheless, the STS Registry reports few redo-SAVRs or TAV-in-SAV, suggesting this is uncommon (3).
With head-to-head trials of SAVR and TAVR, valve failure and durability definitions have become more sophisticated to standardize across studies (14-16). Debate persists on the validity of the VARC-3 definitions as there is little validation or correlation to clinical outcomes. Reintervention after TAVR remains uncommon, with <1% of valves, yet TAVR removal is now the fastest-growing surgical procedure in the STS registry and is associated with high rates of morbidity and mortality (3,17). For redo-SAVR, 30-day mortality has ranged from 2.5–9% (18,19). Surgical removal of a TAV has been associated with much higher risks, in-hospital mortality 11.9%, 30-day 13.1%, and 1-year 28.5% in the EXPLANT-TAVR registry (17).
The reintervention rates for TAVR and SAVR have been low in the randomized controlled trials (4-6). In a pooled analysis of TAVR with Evolut (Medtronic Minneapolis, MN, USA) compared to SAVR, the reintervention rate was similar at 5 years (0.9% vs. 1.5%; P=0.41) (20).
Selecting the right sized valve
Prosthesis-patient mismatch (PPM), or implanting a valve that is too small for a patient’s hemodynamic needs, presents a persistent risk when the implanted valve’s effective orifice area (EOA) is insufficient relative to the patient’s body size, negatively impacting cardiac output requirements (21). A ratio of EOA to body surface area >0.85 cm2/m2 is generally required to avoid unacceptable transvalvular gradients (14,22,23). Severe PPM has been linked to increased short- and long-term mortality (24-30). Furthermore, leaving a patient with a small initial valve, especially with moderate or severe PPM at the time of the initial AVR, leaves minimal options for re-valving, as the initial small platform will constrain the second valve, likely further impacting the hemodynamics of the second valve.
Compared with SAVR, TAVR has been consistently associated with lower rates of PPM, especially with supra-annular self-expanding valves (SEV) (5,31-33). SEVs have generally been associated with lower gradients, larger EOAs, and less PPM than balloon-expandable valves (BEV), particularly supra-annular valves (4,5,34-37). For a patient at risk for severe PPM, a valve with supra-annular leaflets is likely better for reducing PPM risk than an intra-annular or BEV TAVR, which is better than a small surgical valve (38).
Preoperative multidetector cardiac-gated computed tomography (MDCT) helps predict PPM. After determining annular measurements, valve size can be selected, published tables of normal valve EOAs referenced, and an estimate of the risk of PPM for the specific patient can be calculated (38). Unlike SAVR, where the predicted EOA of the surgical valve is fixed, the final size of the TAVR prosthesis is determined by the native annular size. Appropriate treatment strategy with either TAVR or SAVR (with or without root enlargement or replacement) can then be planned to avoid PPM and optimize hemodynamics and durability.
Especially in a young patient with a small aortic annulus undergoing bioprosthetic valve workup, initial treatment options should consider the degree of PPM at index aortic valve intervention and potential size mismatches at redo-valve interventions. During SAVR, aortic root enlargement is a well-described technique that allows implanting surgical aortic valves up to 2–4 sizes larger in some published series (39-42). Root enlargement or replacement procedures may decrease the risk of PPM and early structural valve degeneration at initial SAVR and facilitate future valve-in-valve TAVR options. Of note, a single institution review of TAVR explant patients revealed that an aortic root enlargement procedure was performed in nearly 50% of patients, and the risk of morbidity and mortality was much higher at TAVR explant compared to index SAVR (43).
Simulation for planning the second valve
A cornerstone of failed TAV management is proficiency at “redo-TAVR,” or “TAV-in-TAV” (44). The same simulation for redo-TAVR feasibility can be performed at the initial implant to plan for the most appropriate first and second valves and facilitate lifetime management. In early published series, redo-TAVR was associated with over 85% procedural success, defined as freedom from residual high gradient ≥20 mmHg, regurgitation, and coronary obstruction (which occurred in <1%), and excellent short-term clinical outcomes (45). However, based on the local heart team assessment, 26.8% of failed TAVs had unfavorable anatomy and were not candidates for redo-TAVR. For redo-TAVR, 30-day mortality rates of 0.7–4.6% have been reported (19,45). These cases are often referred to experienced centers, given the non-trivial risk of coronary artery obstruction.
Coronaries are in jeopardy of partial obstruction or complete sequestration when a redo-TAVR stent frame pins open the leaflets of the initial TAV onto the stent frame, forming a covered cylindrical stent graft, creating a “neo-skirt”. Depending on the “neo-skirt” position relative to the sinotubular junction (STJ) and sinus diameters, there may not be sufficient blood flow around the valve and into the coronaries, which could have devastating consequences. This is particularly concerning for supra-annular SEVs with leaflets that can extend to the STJ, but has become more predictable with benchtop measurements and computed tomography (CT) modeling (5,46,47).
To optimize and standardize the redo-TAVR analysis, a step-by-step methodology for evaluating preprocedural CTs for redo-TAVR and determining anatomical feasibility is necessary. Herein, we demonstrate the steps for redo-TAVR with either balloon-expandable SAPIEN valve (S3) (Edwards Lifesciences, Irvine, CA, USA) in Evolut or Evolut-in-Evolut (Figure 1). Notably, the planning for a second valve can be done during pre-TAVR planning for the first valve, and the steps for assessing a virtual second valve are identical to what we describe herein.

Using a failed Evolut as an example, the approach to redo-TAVR CT analysis is based on published in vitro bench testing (47). In the study, S3 valves sized 20 mm, 23 mm, 26 mm, and 29 mm were implanted within 23 mm, 26 mm, 29 mm, and 34 mm Evolut valves, respectively. Analyzing a variety of implantation heights (S3 outflow height aligned with Evolut nodes 4 to 6) demonstrated that the lower implant position of S3-in-Evolut reduces the neo-skirt height while preserving hemodynamic performance despite leaflet overhanging. These values are a reference for CT preplanning (Table 1) (47).
Table 1
Index Evolut | Second TAV | Node level | Neo-skirt height (mm) | Neo-skirt diameter (mm) |
---|---|---|---|---|
23 mm | 20 mm SAPIEN 3 | Node 4 | 16.3 | 19.3 |
Node 5 | 20.7 | 22.9 | ||
Node 6 | 23.9 | 28.2 | ||
23 mm Evolut | 23.3 | |||
26 mm | 23 mm SAPIEN 3 | Node 4 | 17.1 | 23.4 |
Node 5 | 21 | 24.2 | ||
Node 6 | 23.4 | 23.3 | ||
26 mm Evolut | 26.2 | |||
29 mm | 26 mm SAPIEN 3 | Node 4 | 18.3 | 25.8 |
Node 5 | 20.6 | 26.5 | ||
Node 6 | 24.7 | 25.6 | ||
29 mm Evolut | 27.5 | |||
34 mm | 29 mm SAPIEN 3 | Node 4 | 19.9 | 27.4 |
Node 5 | 23 | 28.6 | ||
Node 6 | 27 | 28.5 | ||
34 mm Evolut | 28.9 |
CT analysis may be conducted using several available platforms. 3Mensio (Pie Medical Imaging, Maastricht, The Netherlands) was used to demonstrate a step-by-step CT pre-procedural analysis for redo-TAVR after Evolut. The next generation of 3Mensio, currently in Beta format, is expected to be released in the spring of 2025. Based on native valve anatomy, it can easily simulate a second valve when planning for the first valve. More sophisticated computer simulation using artificial intelligence technology is now approved and may ultimately provide a better way to predict the best valve for a patient’s specific anatomy.
Step 1: select cardiac CT phase
Standard TAVR in native annulus CT analysis is typically done in a systolic phase due to the dynamic nature of the annulus during the cardiac cycle. By contrast, a diastolic phase is preferred for a redo-TAVR workup; the prior implanted TAV is not as dynamic as the native annulus, and the diastolic phase allows better visualization of the TAV commissures. The diastolic phases with the least amount of aortic root motion are selected for accurate measurements.
Step 2: adjust window/level
The window/level setting on the image is adjusted to minimize the degree of blooming artifact in the frame. If the aortic root has contrast opacification, a setting of 1,800/800 is typically used.
Step 3: define and measure the neo-annulus
The neo-annulus is the ventricular edge of the implanted valve at the inflow. The short-axis image of the neo-annulus should include the inferior-most parts of the metal frame, known as “node 0” (Figure 1A). The centerline is set in the middle of the neo-annulus. Dimensions are obtained using polygon tracing to place points in the middle of the metal frame.
Further measurements are assessed to confirm the appropriateness of the second valve size throughout the frame of the initial failed valve. For BEV, an assessment of the midportion or waist and outflow are typically done. For a failed Evolut, measurements are taken at the node level where the second valve is expected to land (see below, Step 6).
Step 4: measure coronary heights
By convention, coronary heights are measured from the basal plane (neo-annulus) to the coronary artery. Publications differ on the superior versus inferior point on the ostium; here, we use the inferior edge. This measurement is made separately for the left and right coronary arteries (Figure 1B).
Step 5: measure sinus heights
Given the risk for sinus sequestration, the sinus height is measured from the neo-annular plane to the point at which the bulge of the sinus tapers inward to become the ascending aorta (Figure 1C).
Step 6: choose appropriate redo valve type and size
Modeling redo-TAVR with S3-in-Evolut is more straightforward and has less risk of coronary flow obstruction and coronary access issues than Evolut-in-Evolut (46). The size of the S3 is based on the neo-annular area and S3 published indications for use, with confirmation at the intended nodal outflow. The original native annular measurements are helpful as well, if available. Notably, it is standard to downsize by at least one size when selecting the S3 for re-valving an Evolut and often necessary to downsize by two valve sizes (i.e., choosing a 23 mm S3 for a second valve in a 29 mm Evolut). Evolut-in-Evolut size is chosen to match the original Evolut’s size in most cases (46).
Step 7: simulate virtual valve implantation depth
The S3 valve shortens from the ventricular side, and aligning the outflow edge with one of the Evolut “nodes” is more practical. Considering the coronary heights and risk plane, nodes 4, 5, or 6 are typically selected as the target of the S3 outflow.
For virtual modeling, the “inflow to inflow” configuration is also reasonable and well supported by benchtop testing, which demonstrates even when the node alignment method (implanting S3 with outflow aligned to node 4 or 5) is used, the final S3 inflow height is just below or just above the Evolut inflow, yielding similar results (47). Benchtop testing also demonstrates that S3 valves do not attain industry-specified nominal measurements when constrained by the Evolut valve (47). Therefore, the virtual valve should be modeled using custom dimensions (Table 1). However, using nominal measurements simulates full expansion of the S3 and may reflect the “worst-case scenario” after balloon post-dilation.
Step 8: determine neo-skirt height
A “neo-skirt” is formed when the valve leaflets are pinned against the stent frame by the second valve, creating a contiguous valve skirt. The neo-skirt height for each combination of Evolut and S3 size was tested and can be used for modeling (Table 1). For Evolut-in-Evolut modeling, the neo-skirt height equals the fully pinned leaflet height. The level of neo-skirt height is annotated on the analysis by placing a line at the appropriate height above the neo-annulus (Figure 1D,1G).
Step 9: assess risk of coronary obstruction
Coronary obstruction can occur with two different mechanisms—direct coronary obstruction and sinus sequestration (48).
The measured coronary height is compared to the neo-skirt height to assess the risk of direct coronary obstruction. If the neo-skirt is below the coronary risk plane, there is no major concern for obstruction. If the neo-skirt height reaches the corresponding coronary height, there may be an obstruction risk, and valve to coronary distance (VTC) should be obtained. The VTC is measured from the valve frame to the proximal-most portion of the coronary artery (Figure 1E,1H). A VTC of <4 mm represents a risk for direct coronary flow obstruction, VTC of ≥4 mm are considered low risk of coronary obstruction but may still have challenging coronary access (Figure 2).
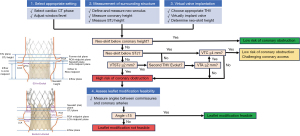
To assess the risk of sinus sequestration, the STJ height is compared to the neo-skirt height. If the neo-skirt height approaches the STJ, there is sinus sequestration risk, so a valve to STJ distance (VTSTJ) should be obtained. The VTSTJ is taken from the valve frame to the aortic wall at the level of the STJ (Figure 1F,1I). A VTSTJ of <2 mm represents a high risk for sinus sequestration. In the case of Evolut-in-Evolut, valve to aorta distance (VTA) is additionally measured if the leaflets extend above the STJ (Figure 1J), and VTA of <2 mm is similarly considered high risk for sinus sequestration (Figure 2).
Step 10: assess commissural alignment
Commissural misalignment (TAV commissure not aligning with the native commissure) can lead to challenging coronary access as the commissure is in front of the coronary ostium. Newer implantation techniques using the cusp overlap view and rotating the delivery catheter have increased commissural alignment to over 95% in native trileaflet valves (49,50), yet with earlier generations, severe malalignment occurred in 20.2% (51). Commercially available S3 valves do not have commissural alignment capabilities, currently under investigation. Assessing commissural alignment before redo-TAVR is especially crucial when the risk of coronary obstruction is high, since misalignment jeopardizes the ability to perform leaflet modification before redo-TAVR (49).
In CT modeling, commissures are typically identified in the diastolic phase when the valve is closed. At the level where the commissures are visible, 360-degree clock measurements are performed, locating the right coronary artery origin, left coronary artery origin, and each commissure (Figure 3). The angles between the coronary arteries and the commissures are calculated. An angle of <15 degrees identifies a commissure in front of a coronary (52)—these cases are likely unsuitable for simple leaflet modification, and alternative measures should be considered (53).
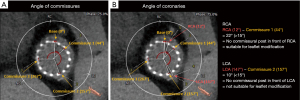
With the increasing number of young and low-risk TAVR patients potentially requiring a second aortic valve intervention, many will prefer redo-TAVR. Planning the second valve during the initial assessment for TAVR versus SAVR is necessary for younger patients with long life expectancy. Comprehensive valve centers must be prepared to optimize the initial valve as well as simulate redo-TAVR and safely offer this operation. The algorithm presented here provides a framework for redo-TAVR workup—which can be done during the planning of the first valve.
As with any virtual valve simulation, in-vivo results will not perfectly match in-situ results; there is no substitute for experienced clinical judgment and operative skills. However, just as low- and high-volume centers have mastered the initial TAVR implantation to produce widespread excellent outcomes, they should be able to simulate the likelihood of a second valve.
Tools and simulation
With the evolution of the technology and a better understanding of valve placement, the number of patients who are anatomical candidates for a second valve is increasing. Leaflet modification strategies (53-55) have been disseminated so that appropriately selected patients who are deemed high risk can safely undergo re-valving, and designated tools are now approved for use (56). Many clinicians question if our assumptions to assess the safety of re-valving are overly conservative and unnecessarily subject patients to higher-risk surgical operations or palliative care. Using artificial intelligence algorithms, such as simulation software like Dasi Simulation, now FDA approved, a much more detailed anatomical assessment can be made in a dynamic aortic root that considers calcium distribution, membranous septum height, coronary access, and can help predict not only the best initial valve, but also simulate the second valve at the time of the initial implant (57). A more sophisticated approach to valve selection for SAVR and TAVR will likely increase the durability of the first valve, making the need for a second valve less likely.
Conclusions
Advanced imaging and simulation tools have changed how we manage severe AS. While no current bioprosthetic valve will have the proven durability of mechanical valves or the Ross procedure, considering patient preferences, heart teams must be equipped with the tools and skills necessary to select the best first valve with the greatest chance of the longest durability while simultaneously accounting for a second valve.
Acknowledgments
None.
Footnote
Funding: None.
Conflicts of Interest: K.J.G. – Abbott, Medtronic, 4C Medical, Ancora; Medtronic employee at the time of publication. P.T.G. – Edwards Lifesciences. The other authors have no conflicts of interest to declare.
Open Access Statement: This is an Open Access article distributed in accordance with the Creative Commons Attribution-NonCommercial-NoDerivs 4.0 International License (CC BY-NC-ND 4.0), which permits the non-commercial replication and distribution of the article with the strict proviso that no changes or edits are made and the original work is properly cited (including links to both the formal publication through the relevant DOI and the license). See: https://creativecommons.org/licenses/by-nc-nd/4.0/.
References
- Otto CM, Nishimura RA, Bonow RO, et al. 2020 ACC/AHA Guideline for the Management of Patients With Valvular Heart Disease: A Report of the American College of Cardiology/American Heart Association Joint Committee on Clinical Practice Guidelines. Circulation 2021;143:e72-e227. [Crossref] [PubMed]
- Vahanian A, Beyersdorf F, Praz F, et al. 2021 ESC/EACTS Guidelines for the management of valvular heart disease. Eur Heart J 2022;43:561-632. [Crossref] [PubMed]
- Carroll JD, Mack MJ, Vemulapalli S, et al. STS-ACC TVT Registry of Transcatheter Aortic Valve Replacement. Ann Thorac Surg 2021;111:701-22. [Crossref] [PubMed]
- Mack MJ, Leon MB, Thourani VH, et al. Transcatheter Aortic-Valve Replacement with a Balloon-Expandable Valve in Low-Risk Patients. N Engl J Med 2019;380:1695-705. [Crossref] [PubMed]
- Popma JJ, Deeb GM, Yakubov SJ, et al. Transcatheter Aortic-Valve Replacement with a Self-Expanding Valve in Low-Risk Patients. N Engl J Med 2019;380:1706-15. [Crossref] [PubMed]
- Thyregod HGH, Jørgensen TH, Ihlemann N, et al. Transcatheter or surgical aortic valve implantation: 10-year outcomes of the NOTION trial. Eur Heart J 2024;45:1116-24. [Crossref] [PubMed]
- Sharma T, Krishnan AM, Lahoud R, et al. National Trends in TAVR and SAVR for Patients With Severe Isolated Aortic Stenosis. J Am Coll Cardiol 2022;80:2054-6. [Crossref] [PubMed]
- Alabbadi S, Malas J, Chen Q, et al. Guidelines vs Practice: Surgical Versus Transcatheter Aortic Valve Replacement in Adults ≤60 Years. Ann Thorac Surg 2025;119:861-9. [Crossref] [PubMed]
- Gupta T, DeVries JT, Gilani F, et al. Temporal Trends in Transcatheter Aortic Valve Replacement for Isolated Severe Aortic Stenosis. J Soc Cardiovasc Angiogr Interv 2024;3:101861. [Crossref] [PubMed]
- Coylewright M, Grubb KJ, Arnold SV, et al. Outcomes of Balloon-Expandable Transcatheter Aortic Valve Replacement in Younger Patients in the Low-Risk Era. JAMA Cardiol 2025;10:127-35. [Crossref] [PubMed]
- Gupta T, Malaisrie SC, Batchelor W, et al. Decision-Making Approach to the Treatment of Young and Low-Risk Patients With Aortic Stenosis. JACC Cardiovasc Interv 2024;17:2455-71. [Crossref] [PubMed]
- Chen S, Pop A, Prasad Dasi L, et al. Lifetime Management for Aortic Stenosis: Strategy and Decision-Making in the Current Era. Ann Thorac Surg 2025;119:296-307. [Crossref] [PubMed]
- Beaver T, Bavaria JE, Griffith B, et al. Seven-year outcomes following aortic valve replacement with a novel tissue bioprosthesis. J Thorac Cardiovasc Surg 2024;168:781-91. [Crossref] [PubMed]
- VARC-3 WRITING COMMITTEE. Valve Academic Research Consortium 3: Updated Endpoint Definitions for Aortic Valve Clinical Research. J Am Coll Cardiol 2021;77:2717-46. [Crossref] [PubMed]
- Capodanno D, Petronio AS, Prendergast B, et al. Standardized definitions of structural deterioration and valve failure in assessing long-term durability of transcatheter and surgical aortic bioprosthetic valves: a consensus statement from the European Association of Percutaneous Cardiovascular Interventions (EAPCI) endorsed by the European Society of Cardiology (ESC) and the European Association for Cardio-Thoracic Surgery (EACTS). Eur J Cardiothorac Surg 2017;52:408-17. [Crossref] [PubMed]
- O’Hair D, Yakubov SJ, Grubb KJ, et al. Structural Valve Deterioration After Self-Expanding Transcatheter or Surgical Aortic Valve Implantation in Patients at Intermediate or High Risk. JAMA Cardiol 2023;8:111-9.
- Bapat VN, Zaid S, Fukuhara S, et al. Surgical Explantation After TAVR Failure: Mid-Term Outcomes From the EXPLANT-TAVR International Registry. JACC Cardiovasc Interv 2021;14:1978-91. [Crossref] [PubMed]
- Hawkins RB, Deeb GM, Sukul D, et al. Redo Surgical Aortic Valve Replacement After Prior Transcatheter Versus Surgical Aortic Valve Replacement. JACC Cardiovasc Interv 2023;16:942-53. [Crossref] [PubMed]
- Thourani VH, Edelman JJ, Meduri CU. TAVR in TAVR: The Future Is Now. J Am Coll Cardiol 2020;75:1894-6. [Crossref] [PubMed]
- Grubb KJ, Lisko JC, O’Hair D, et al. Reinterventions After CoreValve/Evolut Transcatheter or Surgical Aortic Valve Replacement for Treatment of Severe Aortic Stenosis. JACC Cardiovasc Interv 2024;17:1007-16. [Crossref] [PubMed]
- Rahimtoola SH. The problem of valve prosthesis-patient mismatch. Circulation 1978;58:20-4. [Crossref] [PubMed]
- Pibarot P, Dumesnil JG, Jobin J, et al. Usefulness of the indexed effective orifice area at rest in predicting an increase in gradient during maximum exercise in patients with a bioprosthesis in the aortic valve position. Am J Cardiol 1999;83:542-6. [Crossref] [PubMed]
- Pibarot P, Dumesnil JG. Hemodynamic and clinical impact of prosthesis-patient mismatch in the aortic valve position and its prevention. J Am Coll Cardiol 2000;36:1131-41. [Crossref] [PubMed]
- Head SJ, Mokhles MM, Osnabrugge RL, et al. The impact of prosthesis-patient mismatch on long-term survival after aortic valve replacement: a systematic review and meta-analysis of 34 observational studies comprising 27 186 patients with 133 141 patient-years. Eur Heart J 2012;33:1518-29. [Crossref] [PubMed]
- Dayan V, Vignolo G, Soca G, et al. Predictors and Outcomes of Prosthesis-Patient Mismatch After Aortic Valve Replacement. JACC Cardiovasc Imaging 2016;9:924-33. [Crossref] [PubMed]
- Fallon JM, DeSimone JP, Brennan JM, et al. The Incidence and Consequence of Prosthesis-Patient Mismatch After Surgical Aortic Valve Replacement. Ann Thorac Surg 2018;106:14-22. [Crossref] [PubMed]
- Dahlbacka S, Laakso T, Kinnunen EM, et al. Patient-Prosthesis Mismatch Worsens Long-Term Survival: Insights From the FinnValve Registry. Ann Thorac Surg 2021;111:1284-90. [Crossref] [PubMed]
- Sá MP, Jacquemyn X, Van den Eynde J, et al. Impact of Prosthesis-Patient Mismatch After Surgical Aortic Valve Replacement: Systematic Review and Meta-Analysis of Reconstructed Time-to-Event Data of 122 989 Patients With 592 952 Patient-Years. J Am Heart Assoc 2024;13:e033176. [Crossref] [PubMed]
- Dismorr M, Glaser N, Franco-Cereceda A, et al. Effect of Prosthesis-Patient Mismatch on Long-Term Clinical Outcomes After Bioprosthetic Aortic Valve Replacement. J Am Coll Cardiol 2023;81:964-75. [Crossref] [PubMed]
- Sá MP, Jacquemyn X, Van den Eynde J, et al. Impact of Prosthesis-Patient Mismatch After Transcatheter Aortic Valve Replacement: Meta-Analysis of Kaplan-Meier-Derived Individual Patient Data. JACC Cardiovasc Imaging 2023;16:298-310. [Crossref] [PubMed]
- Abbas AE, Ternacle J, Pibarot P, et al. Impact of Flow on Prosthesis-Patient Mismatch Following Transcatheter and Surgical Aortic Valve Replacement. Circ Cardiovasc Imaging 2021;14:e012364. [Crossref] [PubMed]
- Zorn GL 3rd, Little SH, Tadros P, et al. Prosthesis-patient mismatch in high-risk patients with severe aortic stenosis: A randomized trial of a self-expanding prosthesis. J Thorac Cardiovasc Surg 2016;151:1014-22, 1023.e1-3.
- Guimarães L, Voisine P, Mohammadi S, et al. Valve Hemodynamics Following Transcatheter or Surgical Aortic Valve Replacement in Patients With Small Aortic Annulus. Am J Cardiol 2020;125:956-63. [Crossref] [PubMed]
- Pibarot P, Sengupta P, Chandrashekhar Y. Imaging Is the Cornerstone of the Management of Aortic Valve Stenosis. JACC Cardiovasc Imaging 2019;12:220-3. [Crossref] [PubMed]
- Herrmann HC, Mehran R, Blackman DJ, et al. Self-Expanding or Balloon-Expandable TAVR in Patients with a Small Aortic Annulus. N Engl J Med 2024;390:1959-71. [Crossref] [PubMed]
- Leone PP, Regazzoli D, Pagnesi M, et al. Predictors and Clinical Impact of Prosthesis-Patient Mismatch After Self-Expandable TAVR in Small Annuli. JACC Cardiovasc Interv 2021;14:1218-28. [Crossref] [PubMed]
- Leone PP, Scotti A, Ho EC, et al. Prosthesis Tailoring for Patients Undergoing Transcatheter Aortic Valve Implantation. J Clin Med 2023;12:338. [Crossref] [PubMed]
- Grubb KJ, Tom SK, Sultan I, et al. Overcoming prosthesis-patient mismatch with transcatheter aortic valve replacement. Ann Cardiothorac Surg 2024;13:236-43. [Crossref] [PubMed]
- Norton EL, Grubb KJ. Commentary: Aortic root enlargement-optimizing for today and preparing for the future. JTCVS Tech 2022;12:37-8. [Crossref] [PubMed]
- Yazdchi F, Monaghan K, Yang B. Aortic valve replacement with Y-incision/rectangular patch aortic annular enlargement. Indian J Thorac Cardiovasc Surg 2023;39:341-3. [Crossref] [PubMed]
- Tully A, Tom S, Xie J, et al. Evolving computed tomography angiography for aortic valve replacement: Optimizing transcatheter and surgical therapies. J Card Surg 2022;37:4124-32. [Crossref] [PubMed]
- Grubb KJ. Aortic Root Enlargement During Aortic Valve Replacement: Nicks and Manouguian Techniques. Oper Tech Thorac Cardiovasc Surg 2015;20:206-18.
- Nissen AP, Tom SK, Reul RM, et al. Impact of aortic root surgery during transcatheter aortic valve explant. JTCVS Struct Endovasc 2024;1:100002.
- Tarantini G, Sathananthan J, Fabris T, et al. Transcatheter Aortic Valve Replacement in Failed Transcatheter Bioprosthetic Valves. JACC Cardiovasc Interv 2022;15:1777-93. [Crossref] [PubMed]
- Landes U, Webb JG, De Backer O, et al. Repeat Transcatheter Aortic Valve Replacement for Transcatheter Prosthesis Dysfunction. J Am Coll Cardiol 2020;75:1882-93. [Crossref] [PubMed]
- Grubb KJ, Shekiladze N, Spencer J, et al. Feasibility of redo-TAVI in self-expanding Evolut valves: a CT analysis from the Evolut Low Risk Trial substudy. EuroIntervention 2023;19:e330-9. [Crossref] [PubMed]
- Akodad M, Sellers S, Landes U, et al. Balloon-Expandable Valve for Treatment of Evolut Valve Failure: Implications on Neoskirt Height and Leaflet Overhang. JACC Cardiovasc Interv 2022;15:368-77. [Crossref] [PubMed]
- Lederman RJ, Babaliaros VC, Rogers T, et al. Preventing Coronary Obstruction During Transcatheter Aortic Valve Replacement: From Computed Tomography to BASILICA. JACC Cardiovasc Interv 2019;12:1197-216. [Crossref] [PubMed]
- Tang GHL, Amat-Santos IJ, De Backer O, et al. Rationale, Definitions, Techniques, and Outcomes of Commissural Alignment in TAVR: From the ALIGN-TAVR Consortium. JACC Cardiovasc Interv 2022;15:1497-518. [Crossref] [PubMed]
- Vinayak M, Tang GHL, Li K, et al. Commissural vs Coronary Alignment to Avoid Coronary Overlap With THV-Commissure in TAVR: A CT-Simulation Study. JACC Cardiovasc Interv 2024;17:715-26. [Crossref] [PubMed]
- Tang GHL, Zaid S, Fuchs A, et al. Alignment of Transcatheter Aortic-Valve Neo-Commissures (ALIGN TAVR): Impact on Final Valve Orientation and Coronary Artery Overlap. JACC Cardiovasc Interv 2020;13:1030-42. [Crossref] [PubMed]
- Rogers T, Greenspun BC, Weissman G, et al. Feasibility of Coronary Access and Aortic Valve Reintervention in Low-Risk TAVR Patients. JACC Cardiovasc Interv 2020;13:726-35. [Crossref] [PubMed]
- Babaliaros VC, Gleason PT, Xie JX, et al. Toward Transcatheter Leaflet Removal With the CATHEDRAL Procedure: CATHeter Electrosurgical Debulking and RemovAL. JACC Cardiovasc Interv 2022;15:1678-80. [Crossref] [PubMed]
- Khan JM, Greenbaum AB, Babaliaros VC, et al. The BASILICA Trial: Prospective Multicenter Investigation of Intentional Leaflet Laceration to Prevent TAVR Coronary Obstruction. JACC Cardiovasc Interv 2019;12:1240-52. [Crossref] [PubMed]
- Greenbaum AB, Kamioka N, Vavalle JP, et al. Balloon-Assisted BASILICA to Facilitate Redo TAVR. JACC Cardiovasc Interv 2021;14:578-80. [Crossref] [PubMed]
- Haberman D, Chitturi KR, Waksman R. Leaflet modification with the ShortCut™ device to prevent coronary artery obstruction during TAVR. Cardiovasc Revasc Med 2024;65:75-80. [Crossref] [PubMed]
- Holst K, Becker T, Magruder JT, et al. Beyond Static Planning: Computational Predictive Modeling to Avoid Coronary Artery Occlusion in TAVR. Ann Thorac Surg 2025;119:145-51. [Crossref] [PubMed]