Transcatheter versus surgical aortic valve replacement in low- to intermediate-risk patients: a meta-analysis of reconstructed time-to-event data
Introduction
Transcatheter aortic valve replacement (TAVR) has become a well-established alternative to surgical aortic valve replacement (SAVR) for severe symptomatic aortic stenosis (AS) (1,2). Although previous randomized control trials (RCTs) have shown comparable overall mortality for high-, intermediate- and low-risk patient groups (1-8), there remains uncertainty regarding long-term data (9,10). With the exception of the Nordic Aortic Valve Intervention (NOTION) trial, the follow-up periods of no RCTs exceed 5 years (8).
The efficacy of TAVR for low- and intermediate-risk patients remains to be fully elucidated (9,10), and the most recent data from the Evolut Low Risk Trial and the Placement of Aortic Transcatheter Valves 3 (PARTNER 3) trial prompted a reevaluation of the current existing literature (6,7). Given the low event rates in low- and intermediate-risk groups, a meta-analysis would mitigate potential underpowering issues. Furthermore, a previous meta-analysis of RCTs revealed a time-varying association between TAVR and SAVR patients, with a higher mid-term mortality rate with the TAVR group (11). Therefore, a meta-analysis of Kaplan-Meier (KM) derived data, accounting for time-varying effects, holds significance in guiding optimal therapy selection during the decision-making process. Herein, we report a meta-analysis of mid-term outcomes comparing SAVR and TAVR for low- and intermediate-risk patients with severe AS.
Methods
This review was reported according to the Preferred Reporting Items for Systematic Reviews and Meta-Analyses (PRISMA) statement standards (12). Given the nature of our study, Institutional Review Board or Informed Written Consent for Publication was not required. The study protocol was registered on PROSPERO (CRD42023487893).
Literature search strategy
All RCTs comparing TAVR with SAVR for low- and intermediate-risk patients with symptomatic severe AS were identified using a two-level strategy. First, a search of PubMed (MEDLINE), EMBASE, and Cochrane CENTRAL databases was conducted with an experienced medical librarian to identify all the studies published from database inception to November 8th, 2023, that investigated the comparison of TAVR and SAVR in severe symptomatic AS patients. The detailed retrieval strategies are shown in Tables S1-S3. We also searched websites (www.ClinicalTrials.gov, https://www.acc.org, www.escardio.org, https://tctmd.com) for unpublished data.
Eligibility criteria
The included studies met the following criteria: the study design was an RCT, the study population included low- and intermediate-risk patients with symptomatic severe AS, enrolled patients were assigned to the TAVR group or SAVR group, and outcomes included all-cause mortality. Severe AS was defined as AS meeting at least one of the following features: (I) peak velocity 4.0 m/s or greater; (II) mean pressure gradient of 40 mmHg or greater; (III) aortic valve area 1.00 cm2 or less. Low-risk and intermediate-risk patients were defined using the Society of Thoracic Surgeons (STS) risk score of less than 4% for low-risk and between 4% and 8% for intermediate-risk patients. The latest article was included if there were several publications from one trial.
Data extraction and critical appraisal
Relevant studies were identified through a manual search of secondary sources including references of initially identified articles. All references were downloaded for consolidation, elimination of duplicates, and further analyses. Two independent and blinded authors (T.S. and J.S.) reviewed the search results separately to select the studies based on present inclusion and exclusion criteria with a full-text review. Disagreements were resolved by consensus between the two reviewers, with occasional arbitration by a third reviewer (Toshiki Kuno).
Data items
We sought data according to the following PICOS strategy: P (Population), patients with symptomatic severe AS; I (Intervention), TAVR; C (Comparison), SAVR; O (Outcome), all-cause mortality, all-cause mortality or disabling stroke, cardiovascular mortality, rehospitalization due to heart failure, all stroke, disabling stroke, and bioprosthetic valve failure; and S (Study type), RCTs.
Risk of bias in individual studies
Study quality was assessed by two independent and blinded authors (T.S. and J.S.) using the Cochrane Collaboration risk of bias 2.0 (RoB) tool for RCTs (13). Disagreements were resolved by consensus.
Summary measures
The primary outcome of interest was all-cause mortality. The secondary outcomes of interest were a composite outcome (all-cause mortality and disabling stroke), cardiovascular mortality, rehospitalization due to heart failure, all stroke, disabling stroke, and bioprosthetic valve failure. Outcomes including strokes, rehospitalizations, and bioprosthetic valve failure were defined according to the Valve Academic Research Consortium-2 (VARC-2) and VARC-3 endpoint definitions (14,15). The definitions of each outcome are summarized in Table S4 (14,15).
Statistical analysis
We analyzed data from included studies based on the approach demonstrated by Liu and colleagues to obtain reconstructed individual time-to-event data from KM curves (16). First, raw data coordinates, including time and event probability, were extracted from KM curves using WebPlotDigitizer (https://automeris.io/WebPlotDigitizer/) (17). KM curves in each study were reconstructed from data coordinates and the numbers at risk at given time points using the R package “IPDfromKM” (version 0.1.10). Reconstructed individual time-to-event data were used to draw KM curves. To assess the accuracy of the calculated data in comparison to the originally extracted data, root mean square error, mean absolute error, and max absolute error were assessed as measures of precision of the estimation based on Liu’s algorithm (16). The reconstructed KM curves in our study met the recommended thresholds of root mean square error ≤0.05, mean absolute error ≤0.02, and max absolute error ≤0.05.
We merged the reconstructed KM curves of all eligible studies as demonstrated by Liu and colleagues (16). Cox proportional hazard model with stratification by each study was constructed to compare the outcomes between TAVR and SAVR. The proportionality hazards assumption of each Cox model was checked with the Grambsch and Therneau test (18). The Cox hazard models were also constructed from baseline to 1 year and 1 year and beyond (landmark analysis) for each outcome (19). According to the landmark analysis, we defined the following phases: the initial phase (0–1 year; within the first year) and the late phase (1–5 years; beyond the first year).
As a supplement for the Cox proportional hazards model analysis, the restricted mean survival time (RMST) analysis was performed using the survRM2 package (version 1.0.4) in R (20). RMST is the mean duration where patients are free from the outcome, up to a prespecified time point. RMST difference between two treatment groups can be interpreted as the association between treatment and outcome and RMST is considered a robust and interpretable tool. The difference in RMST between the groups for the outcome all-cause mortality shows the lifetime gain or loss associated with the intervention (TAVR) in comparison with the control (SAVR). We set prespecified time points as five years from the initiation of the trial. The analysis was conducted with R Statistical Software (version 4.2.2, Foundation for Statistical Computing, Vienna, Austria).
Additional analyses
Subgroup analyses were conducted by (I) categorizing included studies into TAVRs using balloon-expandable and self-expanding valves; and (II) low- and intermediate-risk studies. Sensitivity analyses were conducted by (I) only incorporating studies with a follow-up period exceeding 1 year; (II) only incorporating studies with >50% use of the current generation of TAVR devices (SAPIEN 3: Edwards Lifesciences, Irvine, CA, USA; and Evolut R, Evolut PRO: Medtronic, Minneapolis, MN, USA). For subgroup analyses, P values for interaction were calculated. When data from multiple studies were unavailable, subgroup and sensitivity analyses were not performed. Substantial heterogeneity was found to be present when the I2 index was over 50%.
Results
Study selection and characteristics
Our analysis included six RCTs (3-8) which enrolled a total of 7,389 patients with severe AS assigned to the TAVR group (n=3,723) and the SAVR group (n=3,666) (Figure S1).
The study profile and patient characteristics are summarized in Table 1. Definitions of symptomatic severe AS for each trial are also summarized in Table 1. To validate the precision of the extracted data from KM curves, we showed reconstructed KM curves for each outcome in Figures S2-S8 for each study.
Table 1
Variables | PARTNER 2A | SURTAVI | UKTAVI | NOTION | PARTNER 3 | Evolut |
---|---|---|---|---|---|---|
Year | 2020 | 2022 | 2022 | 2024 | 2023 | 2023 |
Follow-up (years) | 5 | 5 | 1 | 10 | 5 | 4 |
Definition of severe AS |
Vmax ≥4, PG ≥40 mmHg, AVA ≤0.8 cm2, AVI ≤0.5 cm2/m2 |
Vmax ≥4, PG ≥40 mmHg, AVA ≤0.6 cm2, AVI ≤1.0 cm2/m2 |
N/A | Vmax ≥4, PG ≥40 mmHg, AVA ≤1 cm2, AVI ≤0.6 cm2/m2 |
Vmax ≥4, PG ≥40 mmHg, AVA ≤1 cm2, AVI ≤0.6 cm2/m2 |
Vmax ≥4, PG ≥40 mmHg, AVA ≤1 cm2, AVI ≤0.6 cm2/m2 |
Risk | Intermediate | Intermediate | Low | Low | Low | Low |
Valve type | Balloon | Self | Both | Self | Balloon | Self |
Patients (n) | ||||||
All | 2,032 | 1,746 | 913 | 280 | 950 | 1,468 |
TAVR | 1,011 | 879 | 458 | 145 | 496 | 734 |
SAVR | 1,021 | 867 | 455 | 135 | 454 | 734 |
Age (years) | ||||||
TAVR | 81.5 | 79.9 | 81 | 79.2 | 73.3 | 74 |
SAVR | 81.7 | 79.7 | 81 | 79 | 73.6 | 73.8 |
Male (%) | ||||||
TAVR | 54.2 | 57.6 | 53.9 | 53.8 | 67.5 | 63.2 |
SAVR | 54.8 | 55 | 52.2 | 52.6 | 71.1 | 66.5 |
HTN (%) | ||||||
TAVR | N/A | N/A | 72.1 | 71 | N/A | 84.9 |
SAVR | N/A | N/A | 72.3 | 76.3 | N/A | 82.9 |
DM (%) | ||||||
TAVR | 37.7 | 34.4 | 23.4 | 17.9 | 31.3 | 31.1 |
SAVR | 34.2 | 33.4 | 24.5 | 20.7 | 30.2 | 30.5 |
CAD (%) | ||||||
TAVR | 69.2 | 62.6 | 9.5 | N/A | 27.7 | N/A |
SAVR | 66.5 | 64.2 | 8.9 | N/A | 28 | N/A |
EuroSCORE (%) | ||||||
TAVR | N/A | 11.9 | 2 | N/A | N/A | N/A |
SAVR | N/A | 11.6 | 2.7 | N/A | N/A | N/A |
STS-PROM (%) | ||||||
TAVR | 5.8 | 4.4 | 2.6 | 2.9 | 1.9 | 2 |
SAVR | 5.8 | 4.5 | 2.7 | 3.1 | 1.9 | 1.9 |
PG (mmHg) | ||||||
TAVR | 44.9 | N/A | 73 | N/A | N/A | 47.2 |
SAVR | 44.6 | N/A | 74 | N/A | N/A | 46.7 |
AVA (cm2) | ||||||
TAVR | 0.7 | N/A | 0.7 | N/A | N/A | 0.8 |
SAVR | 0.7 | N/A | 0.7 | N/A | N/A | 0.8 |
PARTNER, Placement of Aortic Transcatheter Valves; SURTAVI, Surgical Replacement and Transcatheter Aortic Valve Implantation; UKTAVI, The UK Transcatheter Aortic Valve Implantation; NOTION, Nordic Aortic Valve Intervention; AS, aortic stenosis; Vmax, peak aortic jet velocity; PG, pressure gradient; AVA, aortic valve area; AVI, aortic valve index; TAVR, transcatheter aortic valve replacement; SAVR, surgical aortic valve replacement; HTN, hypertension; DM, diabetes mellitus; CAD, coronary artery disease; EuroSCORE, European System for Cardiac Operative Risk Evaluation; STS-PROM, Society of Thoracic Surgeons Predicted Risk of Mortality; N/A, not available.
Risk of bias
The risk of bias for each of the included RCT is summarized in Figure S9. One study was at low risk of bias and other five studies were at intermediate risk of bias.
Pooled results from stratified cox proportional hazard model
The all-cause mortality after TAVR compared with SAVR was comparable [hazard ratio (HR), 1.03; 95% confidence interval (CI): 0.93–1.14; P=0.57; six studies] (Figure 1A). The composite outcome of all-cause mortality and disabling stroke was also similar between the two groups (HR, 1.02; 95% CI: 0.92–1.12; P=0.73; four studies) (Figure 1B). The heart failure rehospitalization rates were also similar between the two groups (HR, 1.01; 95% CI: 0.88–1.17; P=0.85; three studies) (Figure 2A). The occurrence of disabling stroke, any stroke, bioprosthetic valve failure, and cardiovascular mortality were also comparable (Figure 2B and Figure S10).
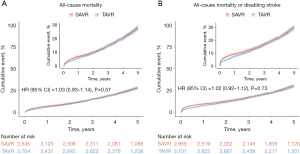
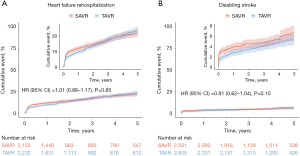
Landmark analyses
Our analysis of 1 year mortality demonstrated lower mortality in the TAVR group in the initial phase (HR, 0.82; 95% CI: 0.68–0.98; P=0.03) (Figure 3A). In contrast, landmark analysis at 1 year demonstrated a higher all-cause mortality in the TAVR group in the late phase (HR, 1.13; 95% CI: 1.01–1.27; P=0.04) (Figure 3A). Similarly to all-cause mortality, the TAVR group was associated with lower composite outcome in the initial phase but higher composite outcome in the late phase (HR, 0.81; 95% CI: 0.68–0.96; P=0.01 and HR, 1.14; 95% CI: 1.01–1.29; P=0.03, respectively) (Figure 3B). The heart failure rehospitalization followed a similar trend, with initially lower but then higher rates in the TAVR group (HR, 0.81; 95% CI: 0.67–0.97; P=0.02, HR, 1.49; 95% CI: 1.17–1.91; P<0.01, respectively) (Figure S11A).
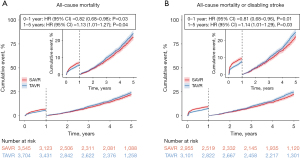
The rates of disabling stroke demonstrated initial favorable results with TAVR and then comparable results in the late phase (HR, 0.72; 95% CI: 0.53–0.97; P=0.03, and HR, 1.11; 95% CI: 0.68–1.82; P=0.68, respectively) (Figure S11B). The rates of any strokes, bioprosthetic valve failure, and cardiovascular mortality were similar in both initial and late phases (Figure S12).
Heterogeneity for each outcome in all phases (0–5, 0–1, 1–5 years) was summarized in Table S5. There were substantial heterogeneities in heart failure rehospitalization for all time periods, in addition to any stroke in the initial phase.
Additional analyses
Subgroup analyses based on the TAVR valve types and the patient risk groups are summarized in Tables S6,S7, demonstrating the HRs and corresponding CIs with SAVR as the control arm. P values for interaction are summarized in Table S8. Subgroup analyses based on the TAVR valve types revealed mostly consistent results as the main analysis (Figure S13). However, the mortality in the late phase was higher with the TAVR group in the subgroup with balloon-expandable TAVR valves, although such a trend was not observed with self-expanding TAVR valves (HR, 1.22; 95% CI: 1.04–1.44; P=0.01, and HR, 1.02; 95% CI: 0.86–1.22; P=0.80, respectively). Subgroup analyses based on the patient risk group were also consistent with the main analysis, except for heart failure rehospitalization (Table S9). The heart failure rehospitalization with the TAVR arm was less frequent than the SAVR arm for low-risk group, while the opposite trend was observed in intermediate-risk groups (HR, 0.66; 95% CI: 0.50–0.88; P<0.01, and HR, 1.19; 95% CI: 1.00–1.42; P<0.05, respectively).
Results from sensitivity analyses based on duration of follow-up periods and newer generation TAVR valves are summarized in Tables S9,S10, demonstrating the HRs and corresponding CIs with SAVR as the control arm. Sensitivity analyses limited to studies with a follow-up period over 1 year demonstrated consistent findings as the main analysis (Figure S14). However, with regard to the 1-year outcome, the preference toward TAVR disappeared. Lastly, sensitivity analyses with new-generation TAVR devices demonstrated lower heart failure rehospitalization in the TAVR group (HR, 0.66; 95% CI: 0.50–0.88; P<0.01) (Figure S15). In the initial phase, favorable outcomes with the TAVR group were observed for rehospitalizations and composite outcome (HR, 0.58; 95% CI: 0.41–0.80; P<0.01, HR, 0.51; 95% CI: 0.31–0.85; P=0.01, respectively).
Grambsch and Therneau test for time-varying effect demonstrated violation of the proportional hazards assumption for the outcomes of all-cause mortality, the composite outcome, rehospitalization, disabling stroke, any stroke, and cardiovascular mortality, which justifies the need for landmark analyses (Table S11). The differences in RMST at 5 years were calculated between SAVR (a reference group) and TAVR. No significant difference was observed for each outcome (Table S12 and Figure S16).
Discussion
We performed a meta-analysis of six RCTs including 7,389 low-/intermediate-risk patients to report the mid-term clinical outcomes. Our analysis demonstrated similar all-cause mortality between TAVR and SAVR. Similarly, the composite outcome of all-cause mortality and disabling stroke was also comparable, as well as heart-failure rehospitalization. In the initial phase (0–1 years), TAVR was associated with favorable all-cause mortality, the composite outcome, disabling stroke, and heart failure rehospitalization. However, in the late phase (1–5 years), TAVR was associated with worse outcomes in all-cause mortality, composite outcome, and heart failure rehospitalization. Although we await the long-term outcomes, our data may be useful in guiding optimal therapy selection during the decision-making process.
The current U.S. and European guidelines both recommend shared decision-making with a Heart Team to opt between TAVR and SAVR (9,10). The U.S. guideline recommends TAVR as an equal alternative to SAVR for patients aged between 65 and 80 years, and TAVR is recommended in preference for patients above age 80 years (9). Meanwhile, the European guideline recommends TAVR for patients over 75 years of age, irrespective of the risk group (10). This discrepancy is due to the absence of robust, definitive long-term data, and the publication of mid-term data for the Evolut and PARTNER 3 trial warrants a reappraisal of the up-to-date data.
Previous meta-analyses demonstrated superior short-term outcomes with the TAVR group for low- and intermediate-risk patients (21-23). Our analysis of 1-year results also demonstrated favorable outcomes with the TAVR arm, congruent to such findings. However, our analysis of mid-term outcomes demonstrated no between-group differences. Additionally, our landmark analysis indicated less favorable outcomes with the TAVR arm in the late phase. This disappearing initial advantage of TAVR coincides with a few meta-analyses employing methods to account for chronological trends, including phase-specific (11) or KM-derived reconstructed time-to-event data approaches (22,24,25). However, such findings in previous reports were likely driven by the high-risk patient groups due to the higher event rates. Our study is the first to demonstrate such a trend specifically for low- and intermediate-risk patients. Notably, our study is the first to also include follow-up data beyond two years for both PARTNER 3 and Evolut Low Risk trials.
The higher mortality initially observed with SAVR can be attributed to its inherently higher procedural invasiveness associated with general anesthesia, full sternotomy, cardioplegic arrest and cardiopulmonary bypass (26). Causes of mortality were previously examined in post-hoc analyses of RCTs concerning self-expandable TAVR valves. Deaths in TAVR arm were primarily linked to procedural technical issues, whereas deaths following SAVR were connected to postoperative complications (26-28). Meanwhile, clinical outcome determinants for long-term outcomes are different, including prosthesis-patient mismatch which can increase mortality for both TAVR (29) and SAVR (30,31) in the long run, paravalvular leaks which are associated with higher risk of all-cause mortality, rehospitalization, and cardiovascular mortality (32), structural valve degeneration and permanent pacemaker implantation which is associated with higher risk of mortality and heart failure-related rehospitalization over time (33). These factors are especially important for low- and intermediate-risk groups, where patients are expected to outlive their implanted valves. The development of new-generation TAVR valves protective against such risks could improve the long-term outcomes. Our sensitivity analysis limited to studies with new-generation TAVR valves demonstrated noninferior survival with a landmark analysis after 1 year, with reduced heart failure rehospitalization. Such results may be influenced by superior fluid dynamics associated with newer valves (34,35).
Despite the growing population receiving TAVRs (36), the literature on cardiac reoperations post-TAVR remains sparse. The incidence of post-TAVR cardiac reoperations significantly increased after the approval of TAVR for low-risk patients by the US Food and Drug Administration in 2019 (37,38). TAVR explants constitute nearly half of post-TAVR reoperations (37) and the proportion of patients belonging to the low-/intermediate-risk groups at the initial TAVR exceeded that of high-risk patients in 2021 (39). This warrants caution, as post-TAVR reoperations are associated with high morbidity and mortality (37,39), and careful consideration is warranted during the initial patient selection process.
Regarding heart failure rehospitalizations, our analysis demonstrated overall similar outcomes between groups. However, our landmark analysis yielded initially lower but eventually higher rates for the TAVR group. Heart failure rehospitalization has been associated with heightened mortality in a post-hoc analysis of the PARTNER trials (40). Interestingly, the subgroup analysis demonstrated the higher late-phase mortality with balloon-expandable valves, while no such trend was observed with self-expanding valves. However, the mortality in the SAVR arm for PARTNER 3 and Evolut Low Risk trials were different (6,7), rendering any direct comparison between the two low-risk trials less definitive. A direct comparison of self-expanding and balloon expandable valves is out of the scope of this study, and our subgroup results are exploratory and only hypothesis-generating at best.
A cautious approach is imperative before extrapolating our findings to clinical practice. It is worth mentioning that a lack of equity was present between the two groups with respect to the frequency of concomitant procedures. As recently mentioned in the joint statement by the STS and European Association for Cardio-Thoracic Surgery, up to 26% of the SAVR group in the PARTNER 3 and Evolut low risk trials underwent concomitant procedures, including concomitant coronary artery bypass graft (CABG) (6,7). These procedures were not only CABGs, but also mitral, tricuspid valve procedures, and interestingly even ascending aortic replacement and septal myectomy in the SAVR arm (6). In the Evolut low risk trial, a notably high mortality rate was observed in the SAVR arm at four years (12.1%) (7). Meanwhile, five-year mortality with isolated SAVR was 7.1% in the STS database report with 42,586 patients, following the same inclusion/exclusion criteria as the PARTNER 3 and Evolut low risk trials (41). In contrast to the SAVR arm, TAVR was almost exclusively an isolated valve procedure, except for few percutaneous coronary interventions. Notably, concomitant SAVR with CABG has been associated with superior outcomes than concomitant TAVR with percutaneous coronary intervention (42).
Additionally, the heterogeneity of implanted prostheses in these RCTs represents an inherent bias. A significant number of TAVR valves were earlier generation prostheses (3,4,8). For both balloon expandable and self-expanding TAVR valves, newer generation devices have been associated with superior outcomes (34,35). In contrast, in the SAVR arm, a number of patients received an externally mounted leaflet prosthesis, which is known to be associated with early bioprosthetic valve failure (43). NOTION and SURTAVI trials, in which approximately 30% of patients in the SAVR arm received these valves, demonstrated strikingly higher bioprosthetic valve failure rates in the SAVR group. When opting for the initial treatment modality for severe, symptomatic AS, a careful patient-tailored approach with consideration to the lifetime management with a Heart Team approach is necessary (9).
An aspect we should keep in mind is that RCTs comparing TAVR with SAVR demonstrate conflicting evidence, particularly in low-risk patients. Jacquemyn et al. (44) recently reevaluated the evidence using trial sequential analysis, balancing type I and II errors, and compared their findings with conventional meta-analysis. Lower-risk RCTs suggested lower death risk on conventional meta-analysis (relative risk, 0.67, 95% CI: 0.47–0.96, P=0.031), but trial sequential analysis indicated potential spurious evidence (P=0.116), necessitating more data for conclusive benefit. For the composite endpoint of death or disabling stroke at 1 year in lower-risk RCTs, TAVR indicated lower risk in conventional meta-analysis (relative risk, 0.68, 95% CI: 0.50–0.93, P=0.014), but trial sequential analysis suggested potential spurious evidence (P=0.053), necessitating more data for conclusive benefit. Follow-up results provided inconclusive evidence for both primary outcomes across risk categories. The authors concluded that conventional meta-analysis methods may have prematurely declared an early reduction of negative outcomes after TAVR when compared with SAVR.
Limitations
This study has several limitations. First, follow-up data beyond 5 years was only available for the NOTION trial (8). Second, not all cases underwent isolated procedures in the included RCTs. Third, the results from subgroup analyses must be interpreted as exploratory outcomes. Fourth, the valve types used in the SURTAVI, PARTNER 2, and NOTION trials are no longer used in the current clinical practice. Furthermore, there was a substantial amount of bias in the included RCTs. A substantial proportion of deviation from randomly assigned treatment, loss to follow-up, and additionally performed procedures were observed in the SAVR group (45).
The frequent loss to follow-up and deviation from assigned treatment in the SAVR arm was markedly higher in comparison to TAVR arm (45,46). In this study, publication bias was not assessed using the funnel plot owning to the insufficient number of included trials in this meta-analysis. Furthermore, incorporating low and intermediate-risk groups may have generated bias in this study. However, as TAVR application expands to these groups, it’s vital to generate relevant evidence, to which this study contributes. Lastly, the SAVR group in the included RCTs contained some portions of patients with concomitant procedures, which could have influenced the results.
Conclusions
This meta-analysis demonstrated that the survival was similar between TAVR and SAVR for low- and intermediate-risk patients with severe symptomatic AS. Our analysis also demonstrated TAVR to be associated with favorable survival in the initial year. However, a landmark analysis indicated suboptimal survival after 1 year in the TAVR arm. Additional RCT results with longer follow-up durations are warranted to confirm this trend.
Acknowledgments
None.
Footnote
Funding: None.
Conflicts of Interest: S.F. is a consultant for Terumo Aortic, Medtronic Inc., Edwards Lifesciences and Artivion. Tsuyoshi Kaneko has received consulting fees from Edwards Lifesciences, Medtronic, 4C Medical, CardioMech, and Cook Medical; and has been a speaker for Abbott and Baylis. The other authors have no conflicts of interest to declare.
Open Access Statement: This is an Open Access article distributed in accordance with the Creative Commons Attribution-NonCommercial-NoDerivs 4.0 International License (CC BY-NC-ND 4.0), which permits the non-commercial replication and distribution of the article with the strict proviso that no changes or edits are made and the original work is properly cited (including links to both the formal publication through the relevant DOI and the license). See: https://creativecommons.org/licenses/by-nc-nd/4.0/.
References
- Mack MJ, Leon MB, Smith CR, et al. 5-year outcomes of transcatheter aortic valve replacement or surgical aortic valve replacement for high surgical risk patients with aortic stenosis (PARTNER 1): a randomised controlled trial. Lancet 2015;385:2477-84. [Crossref] [PubMed]
- Gleason TG, Reardon MJ, Popma JJ, et al. 5-Year Outcomes of Self-Expanding Transcatheter Versus Surgical Aortic Valve Replacement in High-Risk Patients. J Am Coll Cardiol 2018;72:2687-96. [Crossref] [PubMed]
- Van Mieghem NM, Deeb GM, Søndergaard L, et al. Self-expanding Transcatheter vs Surgical Aortic Valve Replacement in Intermediate-Risk Patients: 5-Year Outcomes of the SURTAVI Randomized Clinical Trial. JAMA Cardiol 2022;7:1000-8. [Crossref] [PubMed]
- Makkar RR, Thourani VH, Mack MJ, et al. Five-Year Outcomes of Transcatheter or Surgical Aortic-Valve Replacement. N Engl J Med 2020;382:799-809. [Crossref] [PubMed]
- UK TAVI Trial Investigators. Effect of Transcatheter Aortic Valve Implantation vs Surgical Aortic Valve Replacement on All-Cause Mortality in Patients With Aortic Stenosis: A Randomized Clinical Trial. JAMA 2022;327:1875-87. [Crossref] [PubMed]
- Mack MJ, Leon MB, Thourani VH, et al. Transcatheter Aortic-Valve Replacement in Low-Risk Patients at Five Years. N Engl J Med 2023;389:1949-60. [Crossref] [PubMed]
- Forrest JK, Deeb GM, Yakubov SJ, et al. 4-Year Outcomes of Patients With Aortic Stenosis in the Evolut Low Risk Trial. J Am Coll Cardiol 2023;82:2163-5. [Crossref] [PubMed]
- Thyregod HGH, Jørgensen TH, Ihlemann N, et al. Transcatheter or surgical aortic valve implantation: 10-year outcomes of the NOTION trial. Eur Heart J 2024;45:1116-24. [Crossref] [PubMed]
- Otto CM, Nishimura RA, Bonow RO, et al. 2020 ACC/AHA Guideline for the Management of Patients With Valvular Heart Disease: Executive Summary: A Report of the American College of Cardiology/American Heart Association Joint Committee on Clinical Practice Guidelines. Circulation 2021;143:e35-71. [Crossref] [PubMed]
- Vahanian A, Beyersdorf F, Praz F, et al. 2021 ESC/EACTS Guidelines for the management of valvular heart disease. Eur Heart J 2022;43:561-632. [Crossref] [PubMed]
- Yokoyama Y, Shimoda T, Sloan B, et al. Meta-analysis of phase-specific survival after transcatheter versus surgical aortic valve replacement from randomized control trials. J Thorac Cardiovasc Surg 2024;168:796-808.e27. [Crossref] [PubMed]
- Page MJ, McKenzie JE, Bossuyt PM, et al. The PRISMA 2020 statement: an updated guideline for reporting systematic reviews. BMJ 2021;372: [Crossref] [PubMed]
- Higgins JP, Altman DG, Gøtzsche PC, et al. The Cochrane Collaboration's tool for assessing risk of bias in randomised trials. BMJ 2011;343:d5928. [Crossref] [PubMed]
- Kappetein AP, Head SJ, Généreux P, et al. Updated standardized endpoint definitions for transcatheter aortic valve implantation: the Valve Academic Research Consortium-2 consensus document. Eur Heart J 2012;33:2403-18. [Crossref] [PubMed]
- VARC-3 WRITING COMMITTEE. Valve Academic Research Consortium 3: updated endpoint definitions for aortic valve clinical research. Eur Heart J 2021;42:1825-57. [Crossref] [PubMed]
- Liu N, Zhou Y, Lee JJ. IPDfromKM: reconstruct individual patient data from published Kaplan-Meier survival curves. BMC Med Res Methodol 2021;21:111. [Crossref] [PubMed]
- Drevon D, Fursa SR, Malcolm AL. Intercoder Reliability and Validity of WebPlotDigitizer in Extracting Graphed Data. Behav Modif 2017;41:323-39. [Crossref] [PubMed]
- Shimamura J, Miyamoto Y, Hibino M, et al. Long-Term Outcomes After Hybrid Coronary Revascularization Versus Coronary Artery Bypass Grafting: Meta-Analysis of Kaplan-Meier-Derived Data. Am J Cardiol 2024;212:13-22. [Crossref] [PubMed]
- Gregson J, Sharples L, Stone GW, Burman CF, Öhrn F, Pocock S. Nonproportional Hazards for Time-to-Event Outcomes in Clinical Trials: JACC Review Topic of the Week. J Am Coll Cardiol 2019;74:2102-12. [Crossref] [PubMed]
- McCaw ZR, Yin G, Wei LJ. Using the Restricted Mean Survival Time Difference as an Alternative to the Hazard Ratio for Analyzing Clinical Cardiovascular Studies. Circulation 2019;140:1366-8. [Crossref] [PubMed]
- Kolte D, Vlahakes GJ, Palacios IF, et al. Transcatheter Versus Surgical Aortic Valve Replacement in Low-Risk Patients. J Am Coll Cardiol 2019;74:1532-40. [Crossref] [PubMed]
- Ahmad Y, Howard JP, Arnold AD, et al. Transcatheter versus surgical aortic valve replacement in lower-risk and higher-risk patients: a meta-analysis of randomized trials. Eur Heart J 2023;44:836-52. [Crossref] [PubMed]
- Ando T, Ashraf S, Villablanca P, et al. Meta-Analysis of Effectiveness and Safety of Transcatheter Aortic Valve Implantation Versus Surgical Aortic Valve Replacement in Low-to-Intermediate Surgical Risk Cohort. Am J Cardiol 2019;124:580-5. [Crossref] [PubMed]
- Barili F, Freemantle N, Musumeci F, et al. Five-year outcomes in trials comparing transcatheter aortic valve implantation versus surgical aortic valve replacement: a pooled meta-analysis of reconstructed time-to-event data. Eur J Cardiothorac Surg 2022;61:977-87. [Crossref] [PubMed]
- Sá MP, Jacquemyn X, Van den Eynde J, et al. Midterm Survival of Low-Risk Patients Treated With Transcatheter Versus Surgical Aortic Valve Replacement: Meta-Analysis of Reconstructed Time-to-Event Data. J Am Heart Assoc 2023;12:e030012. [Crossref] [PubMed]
- Gaudiani V, Deeb GM, Popma JJ, et al. Causes of death from the randomized CoreValve US Pivotal High-Risk Trial. J Thorac Cardiovasc Surg 2017;153:1293-1301.e1. [Crossref] [PubMed]
- Amrane H, Deeb GM, Popma JJ, et al. Causes of death in intermediate-risk patients: The Randomized Surgical Replacement and Transcatheter Aortic Valve Implantation Trial. J Thorac Cardiovasc Surg 2019;158:718-728.e3. [Crossref] [PubMed]
- Ramlawi B, Deeb GM, Yakubov SJ, et al. Mechanisms of Death in Low-Risk Patients After Transcatheter or Surgical Aortic Valve Replacement. Cardiovasc Revasc Med 2022;42:1-5. [Crossref] [PubMed]
- Sá MP, Jacquemyn X, Van den Eynde J, et al. Impact of Prosthesis-Patient Mismatch After Transcatheter Aortic Valve Replacement: Meta-Analysis of Kaplan-Meier-Derived Individual Patient Data. JACC Cardiovasc Imaging 2023;16:298-310. [Crossref] [PubMed]
- Sá MP, Jacquemyn X, Van den Eynde J, et al. Impact of Prosthesis-Patient Mismatch After Surgical Aortic Valve Replacement: Systematic Review and Meta-Analysis of Reconstructed Time-to-Event Data of 122 989 Patients With 592 952 Patient-Years. J Am Heart Assoc 2024;13:e033176. [Crossref] [PubMed]
- Sá MPBO, de Carvalho MMB, Sobral Filho DC, et al. Surgical aortic valve replacement and patient-prosthesis mismatch: a meta-analysis of 108 182 patients. Eur J Cardiothorac Surg 2019;56:44-54. [Crossref] [PubMed]
- Sá MP, Jacquemyn X, Van den Eynde J, et al. Impact of Paravalvular Leak on Outcomes After Transcatheter Aortic Valve Implantation: Meta-Analysis of Kaplan-Meier-derived Individual Patient Data. Struct Heart 2023;7:100118. [Crossref] [PubMed]
- Sá MP, Jacquemyn X, Sun T, et al. Late Outcomes of Permanent Pacemaker Implantation After TAVR: Meta-analysis of Reconstructed Time-to-Event Data. J Soc Cardiovasc Angiogr Interv 2022;1:100434. [Crossref] [PubMed]
- Pibarot P, Ternacle J, Jaber WA, et al. Structural Deterioration of Transcatheter Versus Surgical Aortic Valve Bioprostheses in the PARTNER-2 Trial. J Am Coll Cardiol 2020;76:1830-43. [Crossref] [PubMed]
- Giannini C, De Carlo M, Tamburino C, et al. Transcathether aortic valve implantation with the new repositionable self-expandable Evolut R versus CoreValve system: A case-matched comparison. Int J Cardiol 2017;243:126-31. [Crossref] [PubMed]
- Valle JA, Li Z, Kosinski AS, et al. Dissemination of Transcatheter Aortic Valve Replacement in the United States. J Am Coll Cardiol 2021;78:794-806. [Crossref] [PubMed]
- Bowdish ME, Habib RH, Kaneko T, et al. Cardiac Surgery After Transcatheter Aortic Valve Replacement: Trends and Outcomes. Ann Thorac Surg 2024;118:155-62. [Crossref] [PubMed]
- Tang GHL, Zaid S, Kleiman NS, et al. Explant vs Redo-TAVR After Transcatheter Valve Failure: Mid-Term Outcomes From the EXPLANTORREDO-TAVR International Registry. JACC Cardiovasc Interv 2023;16:927-41. [Crossref] [PubMed]
- Fukuhara S, Nguyen CTN, Yang B, et al. Cardiac Reoperations in Patients With Transcatheter Aortic Bioprosthesis. Ann Thorac Surg 2023;116:69-76. [Crossref] [PubMed]
- Huded CP, Arnold SV, Chhatriwalla AK, et al. Rehospitalization Events After Aortic Valve Replacement: Insights From the PARTNER Trial. Circ Cardiovasc Interv 2022;15:e012195. [Crossref] [PubMed]
- Thourani VH, Habib R, Szeto WY, et al. Survival After Surgical Aortic Valve Replacement in Low-Risk Patients: A Contemporary Trial Benchmark. Ann Thorac Surg 2024;117:106-12. [Crossref] [PubMed]
- Sakurai Y, Yokoyama Y, Fukuhara S, et al. Complete transcatheter versus surgical approach to aortic stenosis with coronary artery disease: A systematic review and meta-analysis. J Thorac Cardiovasc Surg 2024;167:1305-1313.e9. [Crossref] [PubMed]
- Yokoyama Y, Sakurai Y, Kuno T, et al. Externally mounted versus internally mounted leaflet aortic bovine pericardial bioprosthesis: meta-analysis. Gen Thorac Cardiovasc Surg 2023;71:207-15. [Crossref] [PubMed]
- Jacquemyn X, Sá MP, Rega F, et al. Transcatheter versus surgical aortic valve replacement for severe aortic valve stenosis: Meta-analysis with trial sequential analysis. J Thorac Cardiovasc Surg 2024; Epub ahead of print. [Crossref]
- Barili F, Brophy JM, Ronco D, et al. Risk of Bias in Randomized Clinical Trials Comparing Transcatheter and Surgical Aortic Valve Replacement: A Systematic Review and Meta-analysis. JAMA Netw Open 2023;6:e2249321. [Crossref] [PubMed]
- Barili F, Anselmi A, Boden WE, et al. Concerns Regarding the Report of 3-Year Outcomes of the Evolut Low Risk Trial. J Am Coll Cardiol 2023;82:e101. [Crossref] [PubMed]