How to advance from minimally invasive coronary artery bypass grafting to totally endoscopic coronary bypass grafting: challenges in Europe versus United States of America
Background
Coronary artery bypass grafting (CABG) is a procedure with a direct effect on life expectancy and quality of life (1). Although, in its early days, the procedure was invasive and required a long recovery time, minimally invasive approaches in the contemporary era have facilitated earlier recovery after surgery. The first minimally invasive left internal mammary artery (LIMA) to left anterior descending artery (LAD) was performed in 1994 by Benetti and Ballester (2), but was a procedure with a steep learning curve, high conversion, and too high complication rates (including post-operative pain) compared to median sternotomy. Techniques and procedures have been optimized since then based on the best knowledge, new techniques and experiences. This evolution leads to a new gold-standard clinical practice and continuously reduce the risk of the surgery. Most recent data underline the importance of the robotic graft harvesting as the graft quality is pivotal in enhancing patency rates and overall outcomes (3). In smaller series, even now thoracoscopic procedures show damage to the internal mammary artery (IMA) in up to 1.8% of cases (4). When the da Vinci robot was introduced in 1998, it allowed surgeons to work in the chest with articulating instruments, minimizing rib spreading, overcoming tremor, and elevating accuracy to the next level. The results of this ‘new’ way of harvesting the IMA were excellent and reiterated in a late European status report (5). Additionally, intra-cardiac procedures showed excellent results with low risk. New robotic platforms are now coming onto the European market and should demonstrate comparable safety and efficacy.
Although the initial dispersion of robotically assisted procedures was rather low and limited to a group of dedicated surgical teams, exponential growth is currently taking place both in USA and Europe. Focus of the initial teams is oriented towards training and education of new surgeons, creating a new safe environment with a lot of background knowledge and support. The available ways of supporting other surgeons can be both live and in a digital environment, but both lead to a higher patient safety as the experienced surgeons co-chair at the operation table.
Medical Device Regulation (MDR) entered into force in May 2021 replacing the Medical Devices Directive (Directive 93/42/EC, ‘MDD’). There was no ‘grandfathering’ (i.e., an automatic acceptance of previously approved devices). In effect, all medical devices were treated as new devices, requiring compliance with a variety of new rules and procedures. For robotic surgical systems and their accessories, when used for interventions in contact with the central circulatory system, this brought the system from class II under the MDD to class III under MDR. This change in risk classification, combined with stricter procedural rules concerning clinical data expectations, led to a general, but not a specific increase in the clinical evidence expectations and as a result, notified bodies (the organizations who assess medical devices for CE-marking) were more likely to request specific clinical investigation data for particular accessories and interventions. The challenge in practice, however, is that it is not possible to know exactly what is required, and this may not be discovered until the manufacturer is undergoing ‘conformity assessment’ (the formal term for the assessment of technical documentation undertaken by a notified body). This likely led to some medical device manufacturers deciding to withdraw devices or accessories from the market, rather than take the risk that their device would be found to have insufficient clinical data following a long and expensive assessment. Some discussions between manufacturers and notified bodies as to the clinical evidence expectations are possible in the form of ’structured dialogue’ (6), however, the type of advice that can be requested or provided is as yet unclear with respect to clinical evidence. European Medicines Agency (EMA) expert panels for medical devices can provide pilot advice to a small number of device developers, however, the pilot will focus on orphan or paediatric devices. European Union (EU) advice structures are in stark contrast to the United States (US) Food and Drug Administration (FDA) system where advice is possible via the Q-Submission programme, where the US FDA can provide clear advice on clinical evidence expectations, with the opportunity to provide consistent advice to developers of similar technologies in a way that the decentralized system of notified bodies cannot. As minimally invasive robotically assisted procedures are estimated to be 3% of the current cardiac procedures, the current benefits for industry were rather small in proceeding to bring the accessories through an assessment, despite the potential benefits for patients [which includes a 20% shorter hospital stay, a shorter intensive care unit (ICU) stay, fewer blood transfusions, and excellent graft patency] (7).
Robotic-assisted (RA) cardiac surgery in the field
Concept
Robotic assistance for coronary surgery was used for the first time in 1999 by Carpentier and Loulmet in Paris (8). It was described as ‘computer-enhanced cardiac surgery’. The terms ‘robotic-assisted’ and ‘computer-enhanced’ covers the topic well; it means the endoscopic procedure is now facilitated by a robotic computer system. This system is a master-slave concept, the instruments that are held by several persons during conventional endoscopic surgery are now held by a digitalized robotic system that mimics the movements made by the surgeon in his console (Figure 1). What is important is that the robotic system does not make any decisions itself and does not leave any implants or marks behind on the patient. It is an external device that facilitates difficult endoscopic surgery by adding more flexibility and accuracy, avoiding tremor and hinge effect.
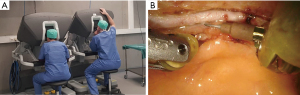
Interaction of the robotic system with the patient
RA cardiac surgery is endoscopic surgery and requires thoracic trocar placement. Depending on the cardiac procedure, 3–4 intercostal trocar placements are made with or without an additional mini-thoracotomy. RA cardiac surgery is less invasive than conventional open cardiac surgery and comes with well-known benefits as shorter intensive care and hospital stay, less blood transfusion, less wound infections (5). The patient is positioned in dorsal decubitus as in conventional surgery. Trocars serve as pivot point and protection for the instruments. The robot is an external system that is positioned next to the operating table (Figure 2). The arms are connected to the trocar to allow an organized movement and articulation of the instruments.
Coronary surgery
The robotic system is used in two types of coronary surgery: RA-minimally invasive direct coronary artery bypass (RA-MIDCAB) grafting and totally endoscopic coronary artery bypass (TECAB) grafting (Figure 3). Both procedures are indicated for surgical coronary bypass grafting, but local legislation and availability of surgical instruments determine which procedure can be performed. The RA-MIDCAB technique is by definition a robotic thoracic procedure, as the robotic assistance is only used in harvesting the IMA on the left side (or bilaterally). After the thoracic phase, a mini-thoracotomy is made to perform the IMA to coronary artery anastomosis. The difference with the TECAB procedure is the possibility of making the coronary anastomosis with the assistance of the robotic system. Both procedures can be performed with or without cardiopulmonary bypass, and all instruments and technologies necessary for this operation have been used and validated for many years. However, due to the absence of agreed upon definitions of minimally invasive CABG, various techniques have developed. Although most of these techniques have contributed to the field, some have misused this term in various ways. It is important to adhere to some elements that define minimal invasive cardiac surgery: minimally invasive cardiothoracic access, off-pump strategy, minimally invasive graft harvesting, anti-inflammatory management, and enhanced recovery after surgery. Maximal compliance with these determinants is encouraged to fall under the definition of minimally invasive coronary surgery, including robotic coronary surgery (9).
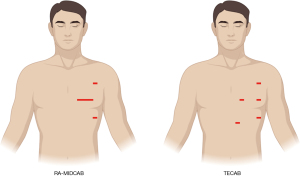
Intra-cardiac surgery
The use of the robotic system in valve surgery mainly involves interventions to the mitral valve (MV). The surgical approach has evolved over the years into a fairly standardized approach. The technique uses four 8 mm robotic ports introduced through the chest wall of the right hemithorax towards the heart. One port is used for the camera and the remaining three for robotic instruments that operate inside the heart. An assistant incision of approximately 1.5–4.0 cm in size is used during the procedure to support the intervention on the valve (Figure 4). The entire procedure can also be performed as a “ports only” procedure, where the assistant incision is replaced by a port with a diameter between 10–12 mm (10).
The main indication for this technique is primary mitral regurgitation in asymptomatic, typically young patients. During the same procedure and with the same approach, a tricuspid valve (TV) repair can also be performed, the left atrial appendage can be closed, and cryoablation of the left or both atria can be performed (11). This approach has also been successfully used for a long time for the correction of congenital heart defects [e.g., patent foramen ovale and atrial septal defect (ASD)] and extirpation of cardiac tumors, such as myxomas (12,13). Recently, the same approach has been successfully used for replacement or repair of the aortic valve. The position of the robotic ports is the same as for MV surgery, the only modification being that the assistant incision must be large enough to allow the artificial valve to be inserted into the chest (14). All of these procedures are by definition intra-cardiac procedures because the robotic instruments directly touch the heart and great vessels.
Clinical evidence on robotic assisted cardiac surgery procedures
Coronary surgery
Surgical revascularization currently accounts for half of the robotic cardiac surgery volume in Europe. Data presented by Cerny et al. (5), covering the years 2016 to 2019, shows that robotic CABG is associated with very low mortality (0.6%) and no strokes. Conversions to larger incisions were performed in 2.6% of patients and the rate of revision for bleeding was 2.1%. Most (98.7%) of these procedures were beating-heart procedures. Cumulative incidence for all robotic procedures indicates that robotic cardiac procedures have a lower in-hospital mortality compared to the predicted EuroSCORE II, mainly driven by the RA-CABG group (Figure 5). Cavallaro et al. (15) studied 2,582 patients undergoing RA-CABG from 2008 to 2010 using the Nationwide Inpatient Sample database, a publicly available database of inpatient hospital care in the USA. The study showed lower stroke and transfusion rates in patients undergoing single-vessel robotic CABG compared to conventional surgery. Multi-vessel operations had similar mortality and cardiovascular complications, regardless of whether a robotic or conventional approach was utilized. A recent 25-year review reported on 1,762 RA-MIDCAB and 1,678 robotic TECAB procedures worldwide was done by Bonatti et al. (16). RA-MIDCAB resulted in 6.6% conversion to larger incisions, 1.9% revision for bleeding, 0.4% stroke, and 0.4% mortality. For robotic TECAB, there was 10.3% conversion to larger incisions, 3.4% revision for bleeding, 1.0% stroke, and 1.3% mortality, but the percentage of multi-vessel bypass surgeries in this cohort was larger. Hospital length of stay was slightly more than 5 days for both methods.
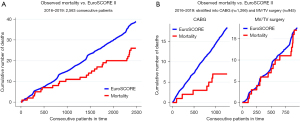
The current European outcomes for robotic CABG, as published in the status report, are comparatively positive (5). The low (2.6%) conversion rate likely reflects a learning curve of the robotic cardiac surgery community and demonstrates that the procedures have become more standardized. This learning curve has also been quantified by Göbölös and coworkers in a review on TECAB (Figure 6) (17). He demonstrated a reduction of the conversion rate from more than 50% to less than 5% over a period of 20 years. In the European status report, very few TECAB procedures were performed, likely due the lack of the Endowrist stabilizer for the new da Vinci X and Xi systems, which were most commonly used in Europe. The revision for bleeding rate of 2.1% was acceptable, but the hospital length of stay of 6.5 days seemed comparable to sternotomy. European healthcare and reimbursement systems, where shorter hospital stays lead to lower remuneration, have probably been a factor in this observation. Early recovery after surgery and return to activities should further be assessed prospectively.
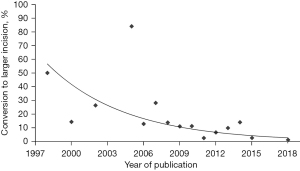
The latest series was published by Lo et al. and al reviewed their 10-year experience involving 562 patients using both mammary and radial grafts operated with RA-MIDCAB. The morbi-mortality rate was low, with conversion to sternotomy of less than 1%. Their long-term results report 5- and 10-year survival of 82% and 65%, respectively. This paper shows that the use of multiple coronary grafts is safe using robotic assistance with the avoidance of sternotomy complications (18).
Intra-cardiac surgery
Robotic MV surgery, including both repair and replacement, accounted for one third of the European volume. The current rates of conversion (2.2%), revision for bleeding (2.3%), and stroke (0.6%) are all in line with published data (5,13,14), while the 1.8% mortality appears higher than that reported in the robotic literature. It needs to be pointed out that combined MV/TV operations (9.6% mortality rate, 5/52) and MV replacement (4.1% mortality rate, 8/197) were included in this analysis, which had a higher mortality rate than isolated TV procedures (0.0% mortality rate, 0/70) and isolated MV repair (MVR) (0.6% mortality rate, 4/626). When considering only the latter, the reported mortality rate can very well compete with results reported from high volume centers in the USA. By comparison, in Chitwood’s series of 540 robotic MV repairs performed at East Carolina University, revision for bleeding, stroke, and mortality occurred in 2.4, 0.6, and 0.4%, respectively. Conversion to sternotomy was necessary in only one patient (0.2%) and the mean hospital stay was 5.6 days (19). In 1,257 patients operated at Emory University School of Medicine, Murphy et al. (20) noted reoperation for bleeding, stroke, and mortality in 2.2%, 0.7%, and 0.6% respectively, with a mean post-operative length of stay of 4.9 days. Urgent conversions were necessary in 1.0%. Gillinov et al. (21) reported an extremely low mortality (0.1%) and stroke rate (1.4%) in the first 1,000 robotic MV patients at the Cleveland Clinic. Conversion to larger incisions occurred in 2.0% (sternotomy) and 2.3% (small thoracotomy), reoperation for bleeding in 2.5%, and median hospital stay was 5 days. Our post-operative hospital stay of 7.5 days exceeded that of USA series and this again is likely a reflection of European reimbursement systems. While robotic MV repair is more commonly performed in the USA, only 1,533 (35.5%) out of 4,322 minimally invasive MV repairs documented in the Society of Thoracic Surgeons (STS) database were carried out using robotic assistance, with only five centers in this analysis performing more than 20 cases per year (22). Data based on recent meta-analyses (23,24) demonstrate that robotic MV procedures offer a lower incidence of postoperative atrial fibrillation, a lower need for transfusions, a shorter stay in the ICU and a shorter overall hospital stay, despite somewhat longer extracorporeal circulation and cardioplegic cardiac arrest times, compared to the classical sternotomy technique. In his meta-analysis including 14 studies and 2,804 patients in the robotic group and 3,537 in the conventional sternotomy group, Williams even demonstrated lower mortality in both matched and unmatched cohorts (23).
The strongest evidence was published in 2024, when Mori et al. (25) performed a retrospective analysis based on data from the STS Adult Cardiac Surgery Database with a total of 7,962 robotic MV procedures. Using propensity matching, they created 6,962 identical pairs for comparing the robotic approach with the sternotomy approach and 5,540 identical pairs for comparing the robotic approach with the mini-thoracotomy approach. Mortality was comparable for all approaches, but the robotic approach demonstrated lower conversion to MV replacement, less need for transfusion, shorter duration of postoperative artificial ventilation, lower incidence of postoperative atrial fibrillation, shorter overall hospital stay, and lower need for hospital readmission compared to both other approaches. Compared to the sternotomy approach, it also demonstrated a lower need for perioperative transfusion.
Robotic MV replacement is technically more complex and more demanding than repair. The major challenge is dealing with calcification and valve suture arrangement with the robotic arms in place. The largest series was reported by Kuo et al. (26) from Tainan and Taipei in Taiwan, including 52 patients who received bioprosthetic replacements with 73% concomitant procedures, such as maze procedures, patent foramen ovale closure, and TV repair. The conversion rate to sternotomy was 1.9% and 1.9% of the patients were re-explored for bleeding. The stroke rate was 1.9%, but there was no in-hospital mortality. Another recent large series of robotic MV replacement by Arslanhan and colleagues reports a mean cardiopulmonary bypass time and cross clamp time were 143±54 and 93±37 minutes, respectively. Mean ICU stay time was 26.5±26.0 hours. Postoperative stroke was observed in one (0.9%) patient and new onset renal failure was observed in two (1.7%) patients. Perioperative and postoperative early mortality was observed in three (2.6%) patients, which was lower than expected. They concluded that robotic MVR can be performed with good early postoperative outcomes (27).
Other procedures eligible for robotic cardiac surgery are atrial septum defect closure, left atrial myxoma resection, epicardial left ventricular lead placement, epicardial ablations (e.g., maze procedure), myocardial bridge division, ventricular tumor resection, and hypertrophic obstructive cardiomyopathy resection. Atrial septum defect closure has been the largest cohort of these other procedures. Multiple case series have been published, all with excellent outcomes and survival (13,28). Regarding the left atrial myxoma resection, robotic surgery is ideally suited for the minimal invasive approach, with the dexterity and flexibility of the instruments allowing for excellent visualization and resection. In the published cases, no conversions nor revisions has been needed (12). Arrhythmia surgery including lead placement and surgical treatment of atrial fibrillation are interesting cases for starting up a robotic program. Small series have shown comparable results to open techniques (29).
Legal aspects towards robotic assisted cardiac surgery
The regulation of robotic surgical systems in Europe
Robotic surgical systems are a type of medical technology that is regulated as a medical device in the EU. In order to be made available in Europe, these systems must comply with the applicable regulations for medical devices. These rules are in the process of significant change in the EU, as the system moves from a directive-based system, with laws initially published in the early 1990s (93/42/EC, ‘MDD’) (5), to the MDR (EU 2017/745 ‘MDR’). The MDR was published in 2017, it came into initial effect in May of 2021 and it has a transitional period that has been extended from 2024 to 2027 for high-risk devices (i.e., class III medical devices). As a result of a series of challenges in complying with the new rules, product withdrawals have been reported across a variety of specialties (7). This may be due to a lack of assessment capacity in notified bodies (the organizations responsible for assessing devices) (8), increased costs and durations of assessments, and a lack of predictability in understanding the type of clinical evidence which is required (9).
When the MDD was first introduced in the 1990s, a compilation of clinical evidence (clinical evaluation) was not required for all devices and was only suggested ‘in particular’ for implantable and class III devices (30). Clinical evidence expectations are markedly different for medical devices when compared to medicines. All medicines require phased clinical trials, whereas medical devices undergo a more procedural assessment known as clinical evaluation, which can utilize data from other devices (demonstrated to be ‘equivalent’), clinical studies, literature reviews and post-market data relating to the use of the device. Under the MDD, there was a much more liberal acceptance of equivalence data, and as a result, it was often possible to avoid the need to conduct pre-market clinical studies by referencing the evidence for another device. MDR changed this by linking the risk classification of a device to a requirement to have ‘clinical investigations’, however, the design or purpose of these investigations is not further described. MDD required a demonstration of safety and ‘performance’. Device performance has been seen as a characteristic of the device alone, to be determined when it is correctly used for its intended purpose on patients, but which is not assessed by a study of patient outcome (31). In other words, if the design engineers tested the device, this could often be taken as sufficient ‘performance’ and the need for a clinical study could often be avoided.
MDR requires that all devices shall be safe and ‘effective’ (32). This is a much stronger clinical evidence requirement, however, it is rarely discussed in guidance documents, which still tend to refer to ‘performance’. Surveys have found that the biggest challenge in preparing clinical evaluations for device developers is determining the “amount of data needed to generate sufficient clinical evidence” (33). Under the MDD rules, which continue to apply during the transition period, robotic surgical systems such as the da Vinci system (Intuitive Surgical, Inc., California, USA) are marketed as intermediate-risk devices (class IIb) for indications including general thoracoscopic surgical procedures (10). As a result of changes in the classification rules in the MDR, Really Simple Syndication (RSS) will in general be upclassified to class III. This upclassification, combined with other changes in the MDR which increases the requirement for clinical investigations for class III devices in particular—has increased the expectation for clinical investigations with respect to individual indications. As the classification rules are based on the timing and anatomical invasiveness of exposure, manufacturers may seek to limit the indications to those which stay within class IIb. As such, the use of these devices with respect to the MDR could become off-label, and the accessories for the higher risk indications may be withdrawn.
In the US, robotic surgical systems are in general class II devices. The classification rules are different between the US and EU. As a result of the classification system in the US, the device can be marketed by following the 510(k) pathway, which allows a clearance based on substantial equivalence to a predicate (i.e., a previously approved device). Robotic surgical systems are medical products, however, the outcomes associated with the use of these products relies significantly on the practice of surgery and the healthcare system in which they are used. The overall responsibilities for the deployment of robotic surgical systems within a hospital therefore requires that device manufacturers, surgeons and health systems each take their responsibility. Surgeons in Europe have a responsibility to ensure that the interventions they conduct are supported by robust clinical evidence. The extent to which this clinical responsibility interacts with the regulatory responsibilities of manufacturers in complying with the MDR is currently unclear, as the clinical evidence requirements are not technology- or intervention-specific. Robotic surgical systems are not without challenges when used for different interventions (11). In some cases, an overall positive benefit/risk is not clear. In the EU, there is high-quality registry data relating to cardiothoracic interventions, however, it is unclear as to whether this is sufficient for notified bodies to approve the device for the MDR.
FDA and RA cardiac surgery
In the US, medical devices are approved by the FDA. Approvals are usually procedure-specific. The da Vinci robot was cleared by the FDA in 1997 for assisting surgery and in 2000 for performing actual surgery. It received clearance for robotically assisted MV surgery in 2002, ASD repair and coronary bypass surgery followed in 2003 and 2004, respectively. These approvals were through so called 510(k) processes and fulfil the requirements for a class II medical device. The different classification and regulatory pathways are important differences between the EU and US systems. There is no guidance for either system concerning type of clinical studies that are necessary to receive regulatory approval and requirements are decided on a case-by-case basis. The current clearance of the da Vinci Surgical robot is “general thoracoscopic and thoracoscopically-assisted cardiotomy procedures”. Under cardiotomy procedures, only MV surgery and coronary artery bypass are included. Any new indication needs to be approved by the FDA in the US meaning procedures like myxoma resection and arrhythmia surgery are by definition off-label uses, and as such they should typically be used under institutional review board (IRB) approval of each hospital and are under the surgeon’s prevue of responsibility to perform them. The FDA is a US federal agency and approvals on a state-level are not possible. The only way to use devices outside official approval is under investigational device exemption (IDE) by the FDA or through IRB approval per hospital. In the US, all medical device manufacturers participate in the FDA MAUDE database which tracks post-market medical device reports regarding safety incidents.
The future of RA cardiac surgery
The future of robotic cardiac surgery holds immense promise. RA cardiac surgery is synonymous with minimally invasive techniques, reducing the need for large incisions, minimizing trauma to the patient, contributing to faster recovery times, reducing pain, and lowering the risks of infection while maintaining (or even improving) patient outcomes. Moreover, the high level of precision offered by robotic cardiac surgery ensures that delicate procedures, through small incisions, are executed with optimum precision. Visualization is paramount in surgery, and robotic systems typically offer an immersive and detailed view of the surgical site. The integration of augmented reality and virtual reality technologies, together with the excellent surgical view, holds immense potential in enhancing the surgeon’s ability to visualize the patient’s anatomy, leading to better identification of critical structures with improved decision-making and surgical planning (e.g., in valve repair, complex congenital defects, etc.). As more data and experience are gathered, robotic systems will become more sophisticated and refined. Machine learning algorithms may be employed to analyze vast amounts of surgical data already available, enabling surgeons to make more informed decisions and customize procedures according to individual patient needs. The use of robotic technology in conjunction with artificial intelligence has the potential to revolutionize the field and further improve patient safety and outcomes.
However, with the introduction of MDR in Europe, patients in Europe may be deprived of these potentially game-changing developments in cardiac surgery, since currently no robotic platform is available for cardiac surgery in Europe due to administrative constraints. The initial research and development work 25 years ago was performed on the da Vinci Si system. The current out phasing of Si and replacement towards Xi systems will make it impossible to use the previously developed Endowrist stabilizer. It will be necessary to re-adapt these tools to the current platforms. To get this work done, engagement of the surgical society as a whole is essential, and a proficiency-based training pathway and learning should be promoted. The procedures, both coronary and MV interventions, are no longer limited to pioneers but are now standardized and safe procedures that need to be taught in a structural way to allow a significant pool of surgical teams to be ready and operate at the highest level. Only then industrial partners will be able to re-engage and will be able to deal with the current MDR constraints and stay economically healthy. The same requirements are applicable to progress from MIDCAB to TECAB, as the knowledge is there but the dispersion and implementation now needs to be improved. The scientific society is best placed to embrace this procedural knowledge, monitor outcomes, and connect with industry to make these crucial steps forward. In recent years, new robotic platforms became available, such as CMR Surgical (United Kingdom), Medicaroid (Japan), SS Innovations (India), Medtronic (USA) and others. These new players might enable the relaunch of robotic cardiac surgery but will benefit from clear accreditation requirements, the cost of accreditation, and timeline.
Conclusions
Robotic cardiac surgery has been present for over 25 years and has demonstrated good early, mid- and long-term results. When the results are compared to conventional ‘open’ surgery, we see the largest progress in terms of blood loss, wound infections, intensive care stay, and total hospital stay while maintaining the standard cardiovascular outcomes. When robotics are compared to other types of minimal invasive surgery, recent evidence also shows better outcomes in favor of RA cardiac surgeries. One may suggest the learning curve for robotics is less difficult compared to thoracoscopic-assisted surgeries and thus lower the threshold to perform robot-assisted cardiac surgery. However, at the moment we are in a legislative framework that creates the absence of certain crucial robotic instruments in Europe. These circumstances have made it difficult to perform robotic MV surgery and pushed the coronary field towards robot-assisted MIDCAB to harvest the internal thoracic arteries but a conventional hand sewn anastomosis instead of TECAB where you can perform the complete procedure without opening the patient’s chest.
Since the da Vinci Si was announced to be outphased, the last centers in the US performing TECAB will have to cease their activities. Together with surgeons and industry, we need to pave a way to keep robotic cardiac surgery at the forefront of innovative cardiovascular medicine and make it available worldwide. Both surgeons and legal authorities must embrace old and new industrial partners to ensure patient safety and optimal outcomes in cardiac surgery. Together, we need to keep working on evidence that supports the use of the Endowrist stabilizer to allow us to perform TECAB. For coronary and intra-cardiac surgery, the techniques have been standardized throughout the years (both for on- and off-pump) with excellent results on medium and long term. All knowledge, evidence and experience combined. RA cardiac surgery seems to be future proofed and is a safe surgical innovation with an added value for the patient. We believe that together with surgeons, societal, industry, and regulatory bodies, RA cardiac surgery can be further developed towards a new standard for select cardiac diseases.
When regulation was revised in the US following the thalidomide tragedy and Kefauver-Harris amendments, it became necessary to demonstrate ‘efficacy’ of medicines for the first time. This led to the establishment of a process with the US FDA to understand which drugs had evidence to support efficacy or not, known as the drug efficacy study implementation (DESI) (34). For medical devices in the EU, we appear to have similarly updated the clinical evidence expectations, however, we have left it to individual developers, companies, and regulators to make a determination on a case-by case basis as to whether the regulatory requirements have been met. In order to bring the regulatory and evidence-based medicine worlds together, we need to develop methods, processes, and specifications to ensure that evidence requirements are clearly defined and communicated to developers, regulatory bodies, and the clinical community in order to ensure that safe and effective interventions can continue and that innovation can be safely introduced.
Acknowledgments
Funding: None.
Footnote
Conflicts of Interest: The authors have no conflicts of interest to declare.
Open Access Statement: This is an Open Access article distributed in accordance with the Creative Commons Attribution-NonCommercial-NoDerivs 4.0 International License (CC BY-NC-ND 4.0), which permits the non-commercial replication and distribution of the article with the strict proviso that no changes or edits are made and the original work is properly cited (including links to both the formal publication through the relevant DOI and the license). See: https://creativecommons.org/licenses/by-nc-nd/4.0/.
References
- van Domburg RT, Kappetein AP, Bogers AJ. The clinical outcome after coronary bypass surgery: a 30-year follow-up study. Eur Heart J 2009;30:453-8. [Crossref] [PubMed]
- Benetti FJ, Ballester C. Use of thoracoscopy and a minimal thoracotomy, in mammary-coronary bypass to left anterior descending artery, without extracorporeal circulation. Experience in 2 cases. J Cardiovasc Surg (Torino) 1995;36:159-61.
- Gaudino M, Audisio K, Rahouma M, et al. Comparison of Long-term Clinical Outcomes of Skeletonized vs Pedicled Internal Thoracic Artery Harvesting Techniques in the Arterial Revascularization Trial. JAMA Cardiol 2021;6:1380-6. [Crossref] [PubMed]
- Duhaylongsod FG, Mayfield WR, Wolf RK. Thoracoscopic harvest of the internal thoracic artery: a multicenter experience in 218 cases. Ann Thorac Surg 1998;66:1012-7. [Crossref] [PubMed]
- Cerny S, Oosterlinck W, Onan B, et al. Robotic Cardiac Surgery in Europe: Status 2020. Front Cardiovasc Med 2022;8:827515. Erratum in: Front Cardiovasc Med 2022;9:870390. [Crossref] [PubMed]
- MDCG 2022-14, Medical Device Coordination Group, MDCG Position Paper - Transition to the MDR and IVDR, Notified Body Capacity and Availability of Medical Devices and IVDs, August 2022, Available online: Https://Health.Ec.Europa.Eu/Document/Download/2db053bc-283c-4d2e-93f4-C3e8032e66da_en?Filename=mdcg_2022-14_en.Pdf
- Algoet M, Verbelen T, Jacobs S, et al. Robot-Assisted MIDCAB Using Bilateral Internal Thoracic Artery: A Propensity Score-Matched Study With OPCAB Patients. Innovations (Phila) 2024;19:184-91. [Crossref] [PubMed]
- Mohr FW, Falk V, Diegeler A, et al. Computer-enhanced coronary artery bypass surgery. J Thorac Cardiovasc Surg 1999;117:1212-4. [Crossref] [PubMed]
- Oosterlinck W, Algoet M, Balkhy HH. Minimally Invasive Coronary Surgery: How Should It Be Defined? Innovations (Phila) 2023;18:22-7. [Crossref] [PubMed]
- Bonatti J, Crailsheim I, Grabenwöger M, et al. Minimally Invasive and Robotic Mitral Valve Surgery: Methods and Outcomes in a 20-Year Review. Innovations (Phila) 2021;16:317-26. [Crossref] [PubMed]
- Palmen M, Navarra E, Bonatti J, et al. Current state of the art and recommendations in robotic mitral valve surgery. Interact Cardiovasc Thorac Surg 2022;35:ivac160. [Crossref] [PubMed]
- Gao C, Yang M, Wang G, et al. Excision of atrial myxoma using robotic technology. J Thorac Cardiovasc Surg 2010;139:1282-5. [Crossref] [PubMed]
- Kadirogullari E, Onan B, Timur B, et al. Transcatheter closure vs totally endoscopic robotic surgery for atrial septal defect closure: A single-center experience. J Card Surg 2020;35:764-71. [Crossref] [PubMed]
- Wei LM, Cook CC, Hayanga JWA, et al. Robotic Aortic Valve Replacement: First 50 Cases. Ann Thorac Surg 2022;114:720-6. [Crossref] [PubMed]
- Cavallaro P, Rhee AJ, Chiang Y, et al. In-hospital mortality and morbidity after robotic coronary artery surgery. J Cardiothorac Vasc Anesth 2015;29:27-31. [Crossref] [PubMed]
- Bonatti J, Wallner S, Crailsheim I, et al. Minimally invasive and robotic coronary artery bypass grafting-a 25-year review. J Thorac Dis 2021;13:1922-44. [Crossref] [PubMed]
- Göbölös L, Ramahi J, Obeso A, et al. Robotic Totally Endoscopic Coronary Artery Bypass Grafting: Systematic Review of Clinical Outcomes from the Past two Decades. Innovations (Phila) 2019;14:5-16. [Crossref] [PubMed]
- Lo CY, Yu CL, Chang Y, et al. Long-term results of robotic-assisted coronary artery bypass grafting with composite arterial grafts for multiple coronary anastomoses: 10-year experience. J Robot Surg 2023;17:63-71. [Crossref] [PubMed]
- Nifong LW, Rodriguez E, Chitwood WR Jr. 540 consecutive robotic mitral valve repairs including concomitant atrial fibrillation cryoablation. Ann Thorac Surg 2012;94:38-42; discussion 43. [Crossref] [PubMed]
- Murphy DA, Moss E, Binongo J, et al. The Expanding Role of Endoscopic Robotics in Mitral Valve Surgery: 1,257 Consecutive Procedures. Ann Thorac Surg 2015;100:1675-81; discussion 1681-2. [Crossref] [PubMed]
- Gillinov AM, Mihaljevic T, Javadikasgari H, et al. Early results of robotically assisted mitral valve surgery: Analysis of the first 1000 cases. J Thorac Cardiovasc Surg 2018;155:82-91.e2. [Crossref] [PubMed]
- Gammie JS, Zhao Y, Peterson ED, et al. J. Maxwell Chamberlain Memorial Paper for adult cardiac surgery. Less-invasive mitral valve operations: trends and outcomes from the Society of Thoracic Surgeons Adult Cardiac Surgery Database. Ann Thorac Surg 2010;90:1401-8, 1410.e1; discussion 1408-10.
- Williams ML, Hwang B, Huang L, et al. Robotic versus conventional sternotomy mitral valve surgery: a systematic review and meta-analysis. Ann Cardiothorac Surg 2022;11:490-503. [Crossref] [PubMed]
- Fatehi Hassanabad A, Nagase FNI, Basha AM, et al. A Systematic Review and Meta-Analysis of Robot-Assisted Mitral Valve Repair. Innovations (Phila) 2022;17:471-81. [Crossref] [PubMed]
- Mori M, Parsons N, Krane M, et al. Robotic Mitral Valve Repair for Degenerative Mitral Regurgitation. Ann Thorac Surg 2024;117:96-104. [Crossref] [PubMed]
- Kuo CC, Chang HH, Hsing CH, et al. Robotic mitral valve replacements with bioprosthetic valves in 52 patients: experience from a tertiary referral hospital. Eur J Cardiothorac Surg 2018;54:853-9. Erratum in: Eur J Cardiothorac Surg 2018;54:967. [Crossref] [PubMed]
- Arslanhan G, Senay S, Kocyigit M, et al. Robotic mitral valve replacement; results from the world's largest series. Ann Cardiothorac Surg 2022;11:533-7. [Crossref] [PubMed]
- Bonaros N, Schachner T, Oehlinger A, et al. Robotically assisted totally endoscopic atrial septal defect repair: insights from operative times, learning curves, and clinical outcome. Ann Thorac Surg 2006;82:687-93. [Crossref] [PubMed]
- Bhatt AG, Steinberg JS. Robotic-Assisted Left Ventricular Lead Placement. Heart Fail Clin 2017;13:93-103. [Crossref] [PubMed]
- The Original MDD 93/42/EEC, Annex X, Section 1.1. Available online: https://www.legislation.gov.uk/eudr/1993/42/annex/X
- Higson GR. Medical Device Safety. The Regulation of Medical Devices for Public Health and Safety. 1st Edition. CRC Press; 2001:158.
- MDR. Annex I - General Safety and Performance Requirements. Available online: https://www.medical-device-regulation.eu/2019/07/23/annex-i-general-safety-and-performance-requirements/
- Kearney B, McDermott O. The Challenges for Manufacturers of the Increased Clinical Evaluation in the European Medical Device Regulations: A Quantitative Study. Ther Innov Regul Sci 2023;57:783-96. [Crossref] [PubMed]
- FDA. Drug Efficacy Study Implementation (DESI). U.S. Food and Drug Administration. (2024). Available online: https://www.fda.gov/drugs/enforcement-activities-fda/drug-efficacy-study-implementation-desi