Adult cardiac transplantation utilizing donors after circulatory death
Introduction
Organ transplantation remains the gold standard and a curative therapy in the treatment of advanced end-stage heart failure (1). There are two approaches to organ donation after patient death which can be medically and legally defined as either (I) irreversible cessation of all brain function, resulting in donation after brain death (DBD) or (II) permanent cessation of circulation, resulting in donation after circulatory death (DCD) (2). Serial neurological examinations are traditionally used to establish brain death with the required documentation of the absence of brain stem reflexes or radiographic demonstration of the absence of brain perfusion (2). Physical examination or intra-arterial pressure monitoring is used to determine the absence of a pulse and confirm circulatory death, confirmed by a certifying physician (2).
Previously, heart utilization for transplantation after DCD donation was rare due to the variable injury with cardiac death, an inability to assess the donor heart’s viability after arrest, and the limitations of cold storage (CS), in contrast to hearts donated by DBD donors where the assessment can occur before circulatory cross-clamp and the injury mitigated by cold preservative solutions (3). However, with the number of listed patients for heart transplantation surpassing the available organs, there has been a significant imbalance of supply and demand, prompting renewed focus on ways to utilize all potential heart donors (4,5). With this renewed focus on donor supply, techniques and technology have been developed to allow the use of DCD heart donors by direct procurement coupled with extracorporeal machine perfusion (MP) and the use of thoracoabdominal normothermic regional perfusion (TA-NRP), a technique which enables resuscitation of the donor heart after circulatory death and its evaluation for transplantation (3).
Since 2014, DCD adult heart transplantation has been steadily increasing in number and across centers (2,6). The United Network of Organ Sharing (UNOS) and organ procurement organizations (OPOs) have formally supported heart transplantation after circulatory death since 2019 (7). DCD donation offers an alternative, often younger, donor pool with a lower degree of comorbidity burden (4).
Pioneering work in Australia and the United Kingdom paved the way for the use of DCD hearts worldwide. Teams at St Vincent’s Hospital in Sydney, Australia, and the Royal Papworth Hospital in Cambridge, England performed early work in this domain, showing equivalent survival outcomes in heart transplantation via DCD versus DBD donors and guiding subsequent work in Spain and the United States (6,8). A multicenter, randomized controlled trial (RCT) of DCD heart donation utilizing direct procurement and MP (Transmedics Organ Care System, Transmedics Andover, MA, USA) in the United States subsequently demonstrated that DCD was non-inferior compared to DBD procurement and evidenced promising short-term outcomes (3). Several single-center and multicenter series utilizing TA-NRP subsequently demonstrated equally effective outcomes in this donor pool in the United States and elsewhere (3,9,10).
It is estimated that in the future, cardiac transplantation via DCD donors could contribute up to a 30% increase in global cardiac transplantations performed (2). This review seeks to provide an updated report of the current knowledge base concerning adult cardiac transplantation utilizing donors after circulatory death and to consider the current challenges and progress required to achieve broader implementation of DCD transplantation.
Approaches
Currently, heart donation via DCD procurement occurs after withdrawal of life sustaining care at the wishes of the donor and/or family after consent and counseling are provided in a controlled and planned process only (Figure 1). Withdrawal of life support treatment (WLST) occurs in a closely monitored, controlled environment with planned procurement in place. After cardiac asystole has occurred, death is confirmed by the certifying physician. From the time of death, there is a required “stand-down” period of typically five minutes based on established ethical and legal requirements of the respective country. This period is needed to detect and avoid procurement in the event of a patient’s spontaneous autoresuscitation after initial asystole. After the stand-down period, two main methods of organ procurement can be used in DCD cardiac transplantation: direct procurement with MP and TA-NRP (11).
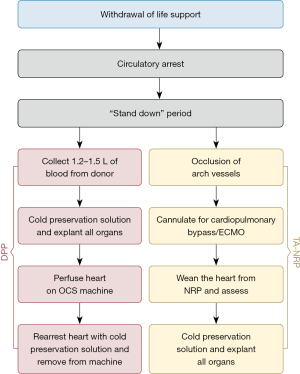
Direct procurement and perfusion (DPP)
In DPP, a sternotomy is performed, the pericardium is then opened, the heart is palpated for coronary artery disease, and the right atrium is cannulated (12,13). Following this instrumentation, 1.2–1.5 L of donor blood is drained to be used for ex-situ reperfusion (14). A bag containing 25,000 units of heparin is used to collect the blood regardless of whether antemortem heparin has been administered. It is also recommended to add tirofiban to the collecting bag (2 mg if platelet count <300,000 and 3 mg if >300,000). Tirofiban is administered to counteract platelet activation and prevent clot formation within the perfusion circuit filters (15). This process usually takes between one and three minutes (12).
After the collection of the donor blood, the cross-clamp is applied and cardioplegia is administered antegrade, whilst ensuring adequate aortic root pressure. In the United Kingdom and Australia, modified St Thomas’ enhanced with erythropoietin and glyceryl trinitrate (known pharmacological conditioning agents) is typically used (2,12). Cardioplegic solution is delivered during organ procurement to try to minimize the effects of ischemia-reperfusion injury and to prevent further depletion of metabolic substrates before reperfusion (12). Cardiectomy then follows (16).
Direct procurement can either use CS or ex-situ normothermic machine perfusion (NMP) using the Organ Care System (OCS) (4,14). The metabolic demands of the heart decrease by 5% with every degree Celsius (°C) cooling. Metabolic demand decreases by 50% at 27 °C compared to 37 °C, with optimal transport of the donor heart occurring at these lower temperatures (14). CS may be used when the donor and recipient are in the same institution, otherwise, the heart is prepared for cannulation onto the OCS heart system for perfusion during transport (16,17).
Upon reanimation, extracorporeal support is gradually reduced. The heart then undergoes ex-situ evaluation (4). Assessment of the viability of the allograft for transplantation is based on a combination of donor and recipient factors such as functional warm ischemic time (fWIT), hemodynamic stability during ex-situ perfusion, and trends in arterial and venous lactate levels, not a single marker or parameter (2). Assessment of the allograft takes place using techniques such as ex-situ coronary angiography which can be used to successfully detect significant stenosis if needed. Due to complex ethics and logistical issues, angiography does not usually take place preretrieval (18). If deemed acceptable and after preparation of the recipient, the heart is cooled to 18 °C during ex-situ perfusion by utilizing an external water heater-cooler (2,4). This is carried out before the administration of the cardioplegic preservation flush, after which the heart can be decannulated and removed from the device, before implantation into the recipient using a standard technique (2).
TA-NRP
The principle underlying TA-NRP involves establishing a bypass circuit using either central or peripheral cannulation, allowing for oxygenation and recirculation of blood to resuscitate the donor organs after excluding circulation to the brain (16). TA-NRP provides perfusion to restore cardiac function, maintain homeostasis and reduce myocardial ischemic injury to maintain the viability of the organs for transplantation (2,9). This resuscitation occurs after the donor has been declared dead and the circulation to the brain has been occluded (16). In the TA-NRP process, the donor undergoes sternotomy; cannulation occurs via the right atrium (venous) and the ascending aorta (arterial) following the clamping of the innominate and left common carotid arteries (5,19). Systemic heparinization may occur antemortem and the heart resumes activity due to re-established coronary perfusion, without perfusion to the brain after the required clamping of the aortic arch vessels (5,7,16). This technique allows the donor heart to be evaluated in situ, more familiarly (12). Current assessment techniques include inspection and echocardiography, but limited data is available on biomarker assessment (9,12).
Inspection of the heart occurs while supported with inotropes, vasopressors or dilators if required, with the aim of maintaining flow at 2.5 to 3.0 L/min and perfusion pressure between 60–80 mmHg (2,19). Support is typically weaned when cardiac contractility improves (19). Assessment of the heart may be done with transesophageal echocardiography, epicardial echocardiography, or hemodynamic assessment directly or via a pulmonary artery catheter placed in the surgical field. Coronary artery angiography and computed tomography scans may be used (2). Electrolyte levels are monitored to check for presence of hypo- or hyperkalemia (19). Normalization of lactate and pH obtained from the aortic root coronary sinus occurs after heartbeat recovery (9). Normothermia for assessment of cardiac function may be beneficial.
Several beneficial drugs can be administered during NRP. These agents may help in mediating oxidative stress and warm ischemia. Specific agents have been detailed in several preclinical studies. Currently, steroids, N-acetylcysteine, erythropoietin, and mannitol are used. These agents have no reports of adverse outcomes on recovered organs, however, there is insufficient clinical data on their benefits (2).
After 30–60 minutes of perfusion, depending on the contractility of the heart and the weaning of inotropes and vasopressors, NRP is then slowly ceased whilst continually assessing the contractility of the myocardium. Once completely weaned off NRP, if there is sufficient cardiac contractility, a decision is made to proceed with donor procurement, or further resuscitation and re-assessment (19). A preservation solution flush is administered to the donor heart and the donor heart can be excised conventionally (12,16). It can then either be placed on an ex vivo NMP or transported with CS (12). The heart is cooled just before implantation (7).
Depending on donor/recipient proximity, donors may be transferred to the recipient institution for procurement (20). An emphasis has been put on co-location of the donor and the recipient to minimize transportation times by institutions such as New York University and Papworth, although this practice is not common outside of these centers. Common practice in the United States, Spain and Belgium is either to have a local team recover for NRP for remote centers or for the recipient centers to send a recovery team (2).
The ideal method of procurement is not defined. While current MP supports long-distance transport with a significant reduction in cold ischemia time (CIT), studies directly comparing techniques have not been performed (19). OCS allows for the heart to remain perfused during dissection and cardiectomy in recipients with complex transplant needs (19).
NMP
NMP involves placing the excised heart into ice slurry and cold saline in preparation for cannulation onto the OCS heart (16). Once cannulated and connected, the donor heart is re-perfused with warm, oxygenated donor blood. Coronary perfusion is achieved in a retrograde fashion with aortic inflow directed against a closed aortic valve (2,16). A vent is placed into the left ventricle via the open left atrium and mitral valve to prevent accumulation of perfusate and distention or air embolism (2). Therefore, the left ventricle is in an empty “resting” state (16). The only venous return to the right atrium is coronary blood flow via the coronary sinus. This enters the right ventricle and then enters the pulmonary artery into the pulmonary artery cannula (2). NMP minimizes ischemic injury compared to direct procurement and static CS alone (12). NMP enables active resuscitation and evaluation of the donor allograft whilst the organ is in transport between the donor and recipient, thereby permitting broader regional sharing (12).
Throughout NMP, serial blood gases are taken from the arterial blood and coronary sinus to guide correction of electrolyte levels, pH, lactate, and oxygen saturation (2,12,16). Perfusate/effluent lactate levels in combination with coronary perfusion are used as markers of the viability of the heart (14). Lactate extraction, indicated by lower venous lactate levels compared to arterial lactate, leading to a gradual decrease in lactate levels over time, suggests organ viability (16). The reliability of lactate as a biomarker is questioned (2). The fWIT may be a more clinically relevant measure of ischemic load (11). Hemodynamic parameters that are monitored during NMP include mean aortic pressure and coronary artery flow, targeted at between 65 and 90 mmHg and 650 and 850 mL/min respectively (16). After stabilization of the heart on the ex-situ device, aortic pressure and flows can be manipulated by adjusting pump flow and administration of epinephrine or adenosine to adjust for lactate levels or other viability concerns (2). Data validating markers and assessments are lacking and often a composite picture is assessed to decide on organ usage upon arrival at the recipient center. Current OCS usage is about 85% of procured organs (3).
Currently, Transmedics OCS (Transmedics Inc., Andover, MA, USA) is the only commercially available platform that offers normothermic, beating-heart perfusion approved in 2022 by the United States Food and Drug Administration (FDA) following the DCD clinical trial. This device provides the potential opportunity to expand the preservation window, facilitate extended criteria donors, and reperfusion of DCD donor hearts (4).
Program logistics
DCD procurement requires significant resources, increasing the cost, complexity, and number of personnel involved; this need poses inherent logistical challenges (21). There is significant variability between centers’ protocols for DCD heart procurement and transplantation due to the enforcement of different regional legislation. These differences are summarized in Table 1. Such differences may be program-based, location-dependent, and country-specific.
Table 1
Characteristics | St Vincent’s (Australia) (6) | Papworth (UK) (22,23) | Liege (Belgium) (24) | Vanderbilt (USA) (10) |
---|---|---|---|---|
Donor age group | Adult <55 years | Adult <55 years | Adult and pediatric | Adult <35 years |
Location of WLST | ICU or Anesthetic Bay | ICU or Anesthetic Bay | Operating room | Not stated |
Ante-mortem interventions | Nil | Nil | Heparin, perfusion cannulas, TOE + Swan-Ganz | Heparin |
Death | Circulatory arrest | Circulatory arrest | Arterial BP <30 mmHg | Circulatory arrest |
Stand-off time | 2–5 min | 5 min | 5 min | 2–5 min |
fWIT | <30 min after SBP <90 mmHg | <30 min after SBP <50 mmHg | Not stated | <35 min after SBP <50 mmHg |
Post-mortem interventions | Cold flush (direct procurement) | Normothermic regional perfusion or cold flush | Normothermic regional perfusion | Normothermic regional perfusion |
Graft retrieval | DP-NMP | DP-NMP, NRP-NMP for distant retrieval NRP-SCS (co-location) | NRP-SCS (co-located, or interhospital transfer) | NRP-SCS for distant retrieval |
Adapted from Scheuer SE, Jansz PC, Macdonald PS. Heart transplantation following donation after circulatory death: Expanding the donor pool. J Heart Lung Transplant 2021;40:882-9 (25). DCD, donation after circulatory death; WLST, withdrawal of life support treatment; ICU, intensive care unit; TOE, transesophageal echocardiogram; BP, blood pressure; fWIT, functional warm ischemic time; SBP, systolic blood pressure; DP-NMP, direct procurement followed by normothermic machine perfusion; NRP-NMP, normothermic regional perfusion followed by normothermic machine perfusion; NRP-SCS, normothermic regional perfusion followed by static cold storage.
Donor ischemic time is directly related to the distance travelled for organ procurement (7). Since DCD hearts are susceptible to ischemic injury, it is recommended that WLST occurs in a location that minimizes the time delay between declaration of death and starting organ procurement (26). The allocation system in the United States, as of 2018, was implemented to broaden organ sharing nationally, using geographic circles of 250, 500, and 1,000 nautical miles from the donor hospital to mark status and priority. This change in allocation resulted in the average distance between donor and recipient hospital increasing from 157 to 279 nautical miles with the CIT being 3–3.4 hours. As a result, there has been a marked increase in donor offers to patients. However, this change forces centers to balance transplant urgency against longer ischemic times, a recognized risk factor for graft dysfunction and decreased posttransplant survival (27).
The SherpaPak™ system (Paragonix Technologies, MA, USA) has recently been shown to improve outcomes with CS. Studies have demonstrated reduced rates of primary graft dysfunction and hence graft injury. Many centers have also stated they use the SherpaPak™ for ischemic times well over four hours (28,29). For centers utilizing the NRP-CS procurement method, this may be an option to safely store the heart to facilitate longer travel distances. The XVIVO perfusion system (XPS Perfusion, Goteborg, Sweden) is another emerging technology being utilized to mitigate the injury of long distances between donors and recipients.
McGiffin and colleagues in Australia and New Zealand have demonstrated that by using hypothermic oxygenated perfusion (HOPE) via the XVIVO heart preservation system, the duration for preserving donor hearts can be safely prolonged, with no risk of primary graft dysfunction, for periods of up to approximately nine hours or more (30). A porcine model has also shown that utilization of oxygenated hypothermic MP with the XVIVO perfusion system resulted in increased biventricular contractility when compared to CS, along with a reduced requirement for inotropic support and fewer signs of myocardial damage (31). It should be noted that currently the XVIVO perfusion system has not yet been approved in the United States (32). However, these findings provide hope that with the adoption of new technologies such as the XVIVO system, longer ischemic times need not translate into inferior outcomes for organ transplant recipients.
Reliable biomarkers and clinical factor scoring systems capable of accurately predicting the rate of progression to circulatory arrest following WLST would aid in improving logistical planning and reducing futile use of resources (2). There has been development of risk scoring systems to predict the trajectory of circulatory collapse in a period that would permit DCD donation, such as the University of Wisconsin donation after cardiac death evaluation tool and the UNOS scoring system (33). In the UNOS criteria, over half of the patients meeting at least one of the UNOS predictors for rapid progression to cardiac arrest went on to experience circulatory death within the first hour of WLST. Sixty-five percent (65%) and 83% of those who met two or three criteria respectively died within one hour (34).
The organization of procurement in DCD donors has challenged established norms. OPOs and the Abdominal and Thoracic teams have developed collaborative processes to allow efficient use and maximize organ use, rather than a win-lose framework. A pre-procurement team call or “huddle” is performed to review the process, experience, and expectations prior to arrival on site. In addition, the use of experienced local teams is emerging as a best practice to allow efficient organization of procurement teams to minimize time to perfusion. Another huddle should take place between teams at the donor hospital before the patient is transported for withdrawal (2).
Costs
Cost is another important consideration surrounding the method of DCD organ procurement. In the US, the use of NRP costs approximately USD $5,000 per donor, whereas the use of MP with the OCS system costs approximately $275,000 for the console and additionally the single-use components cost approximately $38,000–55,000 (17,19). The OCS system also requires maintenance (19). It should also be noted that studies have illustrated that 100% of NRP donated hearts have been used after undergoing assessment and were accepted for transplantation. However, 17% of DPP hearts undergoing perfusion on OCS were rejected for transplantation, each with a potential cost of $114,000 (19). A national service (US) for OCS recovery has been advanced with a direct cost of over $100,000 per episode.
Static CS techniques may eliminate the necessity for costly MP devices such as the Transmedics OCS system and may allow DCD heart transplantation to become more economically viable, however, data is sparse (4).
Regardless of which method is used, significant costs are inherent. The question remains regarding who will pay and whether both options of DCD donation will be equitably available for all necessitating recipients. Particularly in the US, access to transplantation and potential donors may be affected by insurance coverage (17). A cost analysis of both NRP and DPP would be helpful to fully evaluate the financial impact of DCD heart transplantation on individual centers, health systems, and countries.
Decisions
The dead donor rule (DDR)
The DDR was developed to separate organ procurement from the act of killing, ensuring patient protection from undue harm and maintaining the public’s trust in organ donation. The DDR has two components: firstly, organ retrieval should not be the cause of death of the donor individual, secondly, death must be declared before organ procurement (5). This rule is not legal but is an ethical foundation on which the process of organ donation was founded; it is fundamental in the laws and regulations regarding organ and tissue donation (5,17).
There is controversy regarding whether NRP violates the “dead donor rule”. There is no current consensus regarding the use of NRP and varying recommendations regarding NRP-DCD amongst professional societies (4,12). In the US, there is not full acceptance of the NRP technique (12). Discord exists in assessing whether in TA-NRP, the brain has undergone irreversible damage in the five minutes after circulatory death. Occlusion of the head vessels in NRP permits the continuation of cerebral hypoxemia and ischemia, consequently resulting in an irreversible loss of neurologic function despite resuming systemic circulation (12). Therefore, a donor initially declared dead according to circulatory criteria, essentially becomes a donor declared dead as per neurological criteria (12). This method of donation prevents the catecholamine storm and release of other vasoactive substances in response to cerebral ischemia, and also prevents Cushing’s response. Hence, isolation of cerebral circulation improves the quality of the donor organ (17).
Resuming regional thoracic circulation likely does not interfere with the declaration of death any more than resuming regional circulation of the abdomen (2). However, some ethicists and clinicians believe that the DCD donor is not dead but represents the process of death (35). Therefore, they advance that in TA-NRP, death is not able to take its “natural course” in parts of the donor’s body (12). It is also argued that surgically occluding the head vessels in NRP may be insufficient in preventing cerebral reperfusion due to the spinal collateral circulation (4). Therefore, many NRP protocols are dependent on the “permanence” of circulatory arrest after withdrawing life support instead of relying on the “irreversibility” to be compliant with the DDR (12).
Stand-off time
The requisite “stand-off”/“no-touch” time represents the time between cessation of circulation and the formal declaration of death to exclude the likelihood of spontaneous resuscitation (5). During the “no-touch” period, it is imperative not to disturb or influence the process of dying (5). The duration of this period varies between countries and can be anywhere between two to twenty minutes (shown in Table 2) (36). Generally, the absence of circulation for two to five minutes is employed to validate the irreversible loss of circulation, respiration, and brain function (5). One study found that in 480 patients that were declared dead after WLST, of the 14% in which cardiac activity resumed, all events occurred within four minutes and 20 seconds (37). There has been endorsement of the five minute “no-touch” period by the International Society for Heart and Lung Transplantation (ISHLT) (5).
Table 2
Country | “No-touch” period (minutes) |
---|---|
Australia | 2–5 |
Austria | 10 |
Belgium | 5 |
Canada | 5 |
Czech Republic | 5 |
France | 5 |
Ireland | 10 |
Israel | 5 |
Italy | 20 |
Latvia | 5 |
Lithuania | 5 |
Netherlands | 5 |
Norway | 5 |
Poland | 5 |
Portugal | 10 |
Russia | 30 |
Spain | 5 |
Switzerland | 5 |
United Kingdom | 5 |
United States | 2–5 |
Adapted from: Kaffka Genaamd Dengler SE, Vervoorn MT, Brouwer M, et al. Dilemmas concerning heart procurement in controlled donation after circulatory death. Front Cardiovasc Med 2023;10:1225543 (5).
Ischemic time
All DCD procurements initially undergo fWIT/WIT (7,11). The definition of fWIT varies between DCD centers and organ-specific protocols. fWIT is defined commonly as the time from circulatory (systolic blood pressure <50–90 mmHg) and/or respiratory (oxygen saturation <70%) compromise to the delivery of cardioplegia in DPP or extracorporeal reperfusion in NRP (11,12,17). The injury from this global WIT from WLST is unavoidable and occurs when the organ is vulnerable (2,17).
The impact of the obligatory period of warm ischemia following WLST has been a primary concern in DCD donation (2). The WIT has a significant impact in the quality variances seen in hearts in DCD; many potential DCD donors become ineligible because of prolonged fWIT or WIT (12,17). A fWIT<30 min is said to be associated with optimal outcomes (11). Data from preclinical studies suggest that fWITs of more than 30 minutes may result in an injury of the DCD heart that is more likely to result in primary graft failure (2). These donor organs may be less likely to meet viability criteria (2). This interval is unique to DCD organ procurement and has been a key topic in both investigation and ethical conversation (17). Transporting the patient to the operating room or post anesthesia care unit (PACU), prepping and draping the donor, and setting up perfusion before WLST all prevent prolonging fWIT. Such processes have been carried out in Spain, Belgium, and certain USA centers. Another option, when acceptable, is to use shorter “stand-off” periods (16).
Antemortem interventions have also been considered to try and reduce WIT; this includes administering heparin and placing perfusion catheter before WLST. These steps also reduce the risk of post-mortem thrombosis. However, this practice is not always allowed (36). Administering heparin antemortem in DCD donors is an ethically challenging subject, for which there is no international consensus (16). In the UK, antemortem cannulation and heparinization are not permitted. Instead, after a mandatory five-minute “stand-off” period has elapsed, the Papworth technique is used, and heparin is administered directly into the heart (8,23). US centers including The New York University, Langone Health (38), Vanderbilt University Medical Center (Nashville, TN, USA) (10), and Mayo Clinic (Jacksonville) (39) report a similar approach. However, more recently, institutions in Australia and Europe have allowed antemortem interventions during DCD donation (14). The variability regarding this aspect of DCD donation is in part attributed to the complex ethics surrounding DCD transplantation and the scarce scientific evidence supporting the practice of antemortem heparin administration (16). However, preclinical studies in a rodent DCD model have demonstrated that administration of antemortem heparin is related to improved procurement of donor hearts after a DCD protocol (40).
Importantly, the total ischemic insult on a DCD heart refers to both the fWIT and CIT (2). Figure 2 provides an overview of the total ischemic time, fWIT and CIT seen in DPP and TA-NRP. Total ischemic time has been evaluated in univariate and multivariant models of UNOS data and a total ischemic time of <4 hours is associated with the lowest risk. Risk then increases gradually between four and six hours, and increases significantly after exceeding six hours (7).
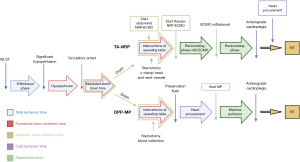
Additionally, it is important to consider asystolic WIT (aWIT), which is the time from asystole to the administration of cardioplegia (16). A report of the outcomes of 74 DCD heart transplant recipients by St Vincent’s has evidenced that increased aWITs correlate with increased requirement for mechanical support in transplant recipients. It is also thought that aWIT may play a significant role in graft dysfunction (41). A separate study also found that aWIT was an independent predictor of primary nonfunction and graft failure after kidney transplantation from DCD donors (42). This aspect of DCD donation requires greater data and analysis to optimize the quality of DCD donor hearts.
DPP versus TA-NRP
The debate between methods of DCD organ procurement primarily originates from different interpretations of circulatory death (5). Advantages of TA-NRP include a shorter fWIT, given that time to open the chest, clamp the head vessels, and initiate bypass is commonly less than six minutes (11). An important benefit of TA-NRP is that it enables in vivo resuscitation and the assessment of the allograft under physiological conditions before retrieval in a familiar fashion. In addition, this technique potentially avoids the cost of MP especially if the heart is determined to be unacceptable for transplantation (14,19). This restoration of native cardiac function facilitates reduction in injury to the myocardium, promotion of energy storage, washing out toxins and re-establishing homeostatic conditions (11,19). The kidneys and liver can provide optimal homeostatic conditions in comparison to the circulated diluted whole blood obtained from the donor in DPP which could affect endothelial cells ultimately resulting in graft dysfunction (5). This benefit allows better selection of donor hearts which may result in a lower rate of complications after transplantation and better outcomes for patients. Additionally, TA-NRP allows perfusion of multiple organs concomitantly which may increase organ recovery and use as well as decrease overall perfusion need (5).
Multiple, albeit small, published series have demonstrated 100% survival at 30 days following DCD transplantation with TA-NRP (8,10,43,44). This information indicates that a larger study could potentially demonstrate increased survival with TA-NRP if the gap between the DPP and TA-NRP is persistent. Furthermore, there is initial evidence to show that DCD hearts recovered via the NRP method have fewer postoperative complications compared to ex-situ perfusion (12). Livers recovered from DCD donors using NRP experience a lower rate of ischemic cholangiopathy (12).
However, ethical concerns in re-establishing a heartbeat and circulation in a person pronounced dead remain significant barriers to wider accepted use of TA-NRP (7,14) despite statements that TA-NRP is ethically justifiable by societies such as the ISHLT in consensus documents (5).
In comparison, DPP is easy to implement into pre-existing procurement procedures since it incorporates techniques involved in DBD cases and does not require a large team as compared to TA-NRP (11). Monitoring the donor graft ex-situ has the potential to allow for graft recovery post warm ischemic injury. Disadvantages of DPP include the requirement to prime the circuit with donor blood (7). In DPP no direct assessment of cardiac function can be made before the removal of the cross-clamp after the heart has been transplanted into the recipient. However, it can be re-perfused with OCS and allows indirect assessment of function (19). The ideal perfusate for NMP of the DCD heart is indicated to be whole blood by preclinical studies. Obtaining whole blood required for perfusate from the deceased donor can take several minutes and may delay the explant of other abdominal organs (2). Blood obtained from the donor may have proinflammatory and prothrombotic factors, high levels of catecholamines, and other drugs that can be detrimental to the procurement of the DCD heart (2,14). Further antithrombotic therapies may be needed to provide optimal ex-situ perfusion. A desirable option is the development of an artificial perfusate with the same desirable benefits of donor blood. This advance would avoid any possible delay in explanting organs in DCD donation (2). Significant cost is added to the DPP method given that MP is routinely used with DPP to transport the heart to the recipient (11). The TransMedics OCS limitations include its expense and its large size which requires the appropriate accommodations for transportation (14).
Both approaches, TA-NRP and DPP are associated with relatively good short and moderate-term outcomes in recipients after DCD compared to DBD (7). Outcomes for DCD heart recipients retrieved via DPP versus NRP have been compared by the Papworth group (8). They found less primary graft dysfunction and increased long-term survival in the NRP group (8). After DCD heart transplantation, any immediate graft dysfunction is commonly transient. Cardiac magnetic resonance (CMR) imaging has been used to follow patients in some centers and has demonstrated complete recovery and no indication of injury pattern (2). Although these single-center experiences are promising, overall outcome data is still limited (2). Since the numbers in current RCTs are small to date, there are currently no comparative studies to advocate for the superiority of one method over the other (14). Overall, DCD transplantation requires larger teams and multiple modalities which is likely to limit the universal adoption of either technique (2).
The advantages and disadvantages of both procurement methods are summarized in Table 3.
Table 3
Heart procurement procedure | Advantages | Disadvantages |
---|---|---|
TA-NRP | • Provides the ideal physiological environment for organ resuscitation | • Ethical debate regarding reestablishing circulation in a donor that has been pronounced dead |
• In vivo assessment of heart function | • Risk of hypoxia | |
• Shorter fWIT | • Medical interventions required to enable reanimation and for functional assessment | |
• Increased survival | ||
• Fewer postoperative complications | ||
DPP | • Easy to implement into pre-existing procurement procedures | • Requirement to prime the circuit with donor blood |
• Ex vivo functional assessment | ||
• Does not require a large team | • Significant cost of OCS | |
• Ex situ assessment of the heart allow for graft recovery post warm ischemic injury | • Logistic challenges in transportation due to the large equipment size | |
• Decreased geographical limitations since travel times can be expanded | • Requires significant technical expertise and training required to set up and operate MP | |
• CS only suitable for colocalized donor and recipient at the same institution |
DCD, donation after circulatory death; TA-NRP, thoraco-abdominal regional perfusion; fWIT, functional warm ischemic time; DPP, direct procurement and perfusion; OCS, Organ Care System; MP, machine perfusion; CS, cold storage.
Perfusion
Given the heart’s high metabolic demands relative to other organs, only blood based perfusate will enable it to remain in an aerobic condition at normothermia (14). There has been evidence from preclinical studies that altering the composition and temperature of the initial solution used to flush will protect the DCD heart from reperfusion injury as a result of ischemia (2). There are new non-blood based perfusates as impermeant, for example, modified UW-MPS which incorporates polyethylene glycol (PEG-20K). This technique has shown positive results in preserving DCD kidneys and livers. Another low-pressure perfusion solution has also shown promising results in DCD animal and human hearts (non-transplanted) (14).
In the future, an asanguineous perfusion system, either delivered by gravity or MP, compact enough for transport and economically feasible, will help propel the ex-situ perfusion and transportation of DCD hearts (14,17). There are five devices currently being developed with results published from using them in animal heart experiments and one study in DCD human heart transplantation. These advances in ex-situ perfusion methods will improve markers of viability of the myocardium. There is potential for DCD hearts to be both resuscitated from ischemic injury and protected from immunologic injury. Currently, in lung transplantation, ex-situ platforms have achieved this such as the Toronto EVLP System (Lung Bioengineering, a division of United Therapeutics Corp, Silver Spring, MD, USA). They encompass antibiotic therapy, inflammation modulation, exposure to anti-ischemic metabolites, vasodilators, ischemic postconditioning, edema reduction with hyperosmolar solutions, and immunomodulation via viral transfection (14). Further investigation is necessary to ascertain the optimal solution for DPP and to identify the most favorable conditions for perfusing blood in both DPP and NRP.
Conclusions
DCD donation presents both opportunities and challenges in the realm of heart transplantation. Its emergence holds promise for narrowing the gap between patients in need of organs and the available donor pool. While short and intermediate outcomes of DCD transplantation currently match those of DBD transplantation, ongoing vigilance is vital for monitoring the long-term prospects of these patients, especially concerning their susceptibility to coronary allograft vasculopathy compared to recipients of DBD hearts.
The influence of DCD procurement methods on transplantation probability and outcome disparities due to limited sample sizes are areas that warrant further investigation. The adoption of NMP also introduces financial and logistical considerations, including the transportation and continual monitoring of OCS hearts, raising the question of whether TA-NRP should become the preferred approach to DCD procurement.
The rapid emergence of DCD use has allowed heart transplant volume to increase worldwide. Data collection and process standardization have been lacking due to the uneven and at times rapid adoption of these techniques. Long-term outcomes and best practices remain to be defined and are important considerations in the wider use of these techniques in a broad selection of patients to understand best use and practice moving forward.
Expanding DCD donation entails substantial resource allocation, coordination efforts, and training initiatives. Currently, there exists considerable variability in regulatory frameworks, professional guidelines, and documented practices related to the DCD procurement process. Moving forward, standardization is imperative, particularly concerning aspects such as “stand-off” time, WIT, and perfusate composition. Addressing these disparities and establishing a universally endorsed recommendation are essential steps toward wider DCD implementation.
Acknowledgments
Funding: None.
Footnote
Conflicts of Interest: H.C. has been a speaker for Paragonix and Abbott. The other authors have no conflicts of interest to declare.
Open Access Statement: This is an Open Access article distributed in accordance with the Creative Commons Attribution-NonCommercial-NoDerivs 4.0 International License (CC BY-NC-ND 4.0), which permits the non-commercial replication and distribution of the article with the strict proviso that no changes or edits are made and the original work is properly cited (including links to both the formal publication through the relevant DOI and the license). See: https://creativecommons.org/licenses/by-nc-nd/4.0/.
References
- Beaupré RA, Morgan JA. Donation After Cardiac Death: A Necessary Expansion for Heart Transplantation. Semin Thorac Cardiovasc Surg 2019;31:721-5. [Crossref] [PubMed]
- Schroder JN, Scheuer S, Catarino P, et al. The American Association for Thoracic Surgery 2023 Expert Consensus Document: Adult cardiac transplantation utilizing donors after circulatory death. J Thorac Cardiovasc Surg 2023;166:856-869.e5. [Crossref] [PubMed]
- Schroder JN, Patel CB, DeVore AD, et al. Transplantation Outcomes with Donor Hearts after Circulatory Death. N Engl J Med 2023;388:2121-31. [Crossref] [PubMed]
- Hess NR, Ziegler LA, Kaczorowski DJ. Heart Donation and Preservation: Historical Perspectives, Current Technologies, and Future Directions. J Clin Med 2022;11:5762. [Crossref] [PubMed]
- Kaffka Genaamd Dengler SE, Vervoorn MT, Brouwer M, et al. Dilemmas concerning heart procurement in controlled donation after circulatory death. Front Cardiovasc Med 2023;10:1225543. [Crossref] [PubMed]
- Chew HC, Iyer A, Connellan M, et al. Outcomes of Donation After Circulatory Death Heart Transplantation in Australia. J Am Coll Cardiol 2019;73:1447-59. [Crossref] [PubMed]
- Hendren NS, Truby LK, Farr M. Donation after circulatory determination of death in heart transplant: impact on current and future allocation policy. Curr Opin Cardiol 2023;38:124-9. [Crossref] [PubMed]
- Messer S, Cernic S, Page A, et al. A 5-year single-center early experience of heart transplantation from donation after circulatory-determined death donors. J Heart Lung Transplant 2020;39:1463-75. [Crossref] [PubMed]
- Nistal JF, Cobo M, Larraz E, et al. Heart transplantation from controlled donation after circulatory death using thoracoabdominal normothermic regional perfusion and cold storage. J Card Surg 2021;36:3421-4. [Crossref] [PubMed]
- Hoffman JRH, McMaster WG, Rali AS, et al. Early US experience with cardiac donation after circulatory death (DCD) using normothermic regional perfusion. J Heart Lung Transplant 2021;40:1408-18. [Crossref] [PubMed]
- Srivastava PK, Kittleson MM. Modern advances in heart transplantation. Prog Cardiovasc Dis 2024;82:147-56. [Crossref] [PubMed]
- Truby LK, Casalinova S, Patel CB, et al. Donation After Circulatory Death in Heart Transplantation: History, Outcomes, Clinical Challenges, and Opportunities to Expand the Donor Pool. J Card Fail 2022;28:1456-63. [Crossref] [PubMed]
- Page A, Messer S, Large SR. Heart transplantation from donation after circulatory determined death. Ann Cardiothorac Surg 2018;7:75-81. [Crossref] [PubMed]
- Quader M, Toldo S, Chen Q, et al. Heart transplantation from donation after circulatory death donors: Present and future. J Card Surg 2020;35:875-85. [Crossref] [PubMed]
- Scheuer SE, Soto C, Joseph J, et al. Platelet activation after withdrawal of life support in donation after circulatory death donors. J Heart Lung Transplant 2020;39:1494-6. [Crossref] [PubMed]
- Joshi Y, Villanueva J, Gao L, et al. Donation After Circulatory Death: A New Frontier. Curr Cardiol Rep 2022;24:1973-81. [Crossref] [PubMed]
- Fedson S. Heart transplant donation after circulatory death: current status and implications. Curr Opin Cardiol 2024;39:128-34. [Crossref] [PubMed]
- Nadel J, Scheuer S, Kathir K, et al. Successful transplantation of high-risk cardiac allografts from DCD donors following ex vivo coronary angiography. J Heart Lung Transplant 2020;39:1496-9. [Crossref] [PubMed]
- Alamouti-Fard E, Garg P, Wadiwala IJ, et al. Normothermic Regional Perfusion is an Emerging Cost-Effective Alternative in Donation After Circulatory Death (DCD) in Heart Transplantation. Cureus 2022;14:e26437. [Crossref] [PubMed]
- Hosseini M, Stawiarski KM, Ramakrishna H. Donation After Circulatory Death (DCD) Heart Transplantation- Analysis of Recent Data. J Cardiothorac Vasc Anesth 2023;37:1801-3. [Crossref] [PubMed]
- Dhital K, Ludhani P, Scheuer S, et al. DCD donations and outcomes of heart transplantation: the Australian experience. Indian J Thorac Cardiovasc Surg 2020;36:224-32. [Crossref] [PubMed]
- Messer S, Page A, Colah S, et al. Human heart transplantation from donation after circulatory-determined death donors using normothermic regional perfusion and cold storage. J Heart Lung Transplant 2018;37:865-9. [Crossref] [PubMed]
- Messer SJ, Axell RG, Colah S, et al. Functional assessment and transplantation of the donor heart after circulatory death. J Heart Lung Transplant 2016;35:1443-52. [Crossref] [PubMed]
- Tchana-Sato V, Ledoux D, Detry O, et al. Successful clinical transplantation of hearts donated after circulatory death using normothermic regional perfusion. J Heart Lung Transplant 2019;38:593-8. [Crossref] [PubMed]
- Scheuer SE, Jansz PC, Macdonald PS. Heart transplantation following donation after circulatory death: Expanding the donor pool. J Heart Lung Transplant 2021;40:882-9. [Crossref] [PubMed]
- Cao Y, Shahrestani S, Chew HC, et al. Donation After Circulatory Death for Liver Transplantation: A Meta-Analysis on the Location of Life Support Withdrawal Affecting Outcomes. Transplantation 2016;100:1513-24. [Crossref] [PubMed]
- Kilic A, Mathier MA, Hickey GW, et al. Evolving Trends in Adult Heart Transplant With the 2018 Heart Allocation Policy Change. JAMA Cardiol 2021;6:159-67. [Crossref] [PubMed]
- Leacche M, Philpott J, Pham S, et al. US multi-center analysis of the Global Utilization and Registry Database for Improved Heart Preservation (GUARDIAN) registry: 1-year transplant survival analysis. J Heart Lung Transplant 2022;41:S30-1.
- Radakovic D, Karimli S, Penov K, et al. First clinical experience with the novel cold storage SherpaPak™ system for donor heart transportation. J Thorac Dis 2020;12:7227-35. [Crossref] [PubMed]
- McGiffin DC, Kure CE, Macdonald PS, et al. Hypothermic oxygenated perfusion (HOPE) safely and effectively extends acceptable donor heart preservation times: Results of the Australian and New Zealand trial. J Heart Lung Transplant 2024;43:485-95. [Crossref] [PubMed]
- Moeslund N, Ertugrul IA, Hu MA, et al. Ex-situ oxygenated hypothermic machine perfusion in donation after circulatory death heart transplantation following either direct procurement or in-situ normothermic regional perfusion. J Heart Lung Transplant 2023;42:730-40. [Crossref] [PubMed]
- Kothari P. Ex-Vivo Preservation of Heart Allografts-An Overview of the Current State. J Cardiovasc Dev Dis 2023;10:105. [Crossref] [PubMed]
- Lewis J, Peltier J, Nelson H, et al. Development of the University of Wisconsin donation After Cardiac Death Evaluation Tool. Prog Transplant 2003;13:265-73. [Crossref] [PubMed]
- DeVita MA, Brooks MM, Zawistowski C, et al. Donors after cardiac death: validation of identification criteria (DVIC) study for predictors of rapid death. Am J Transplant 2008;8:432-41. [Crossref] [PubMed]
- Morrissey PE, Monaco AP. Donation after circulatory death: current practices, ongoing challenges, and potential improvements. Transplantation 2014;97:258-64. [Crossref] [PubMed]
- Crespo-Leiro MG, Costanzo MR, Gustafsson F, et al. Heart transplantation: focus on donor recovery strategies, left ventricular assist devices, and novel therapies. Eur Heart J 2022;43:2237-46. [Crossref] [PubMed]
- Dhanani S, Hornby L, van Beinum A, et al. Resumption of Cardiac Activity after Withdrawal of Life-Sustaining Measures. N Engl J Med 2021;384:345-52. [Crossref] [PubMed]
- Ngai J, Masuno K, Moazami N. Anesthetic Considerations During Heart Transplantation Using Donation After Circulatory Death. J Cardiothorac Vasc Anesth 2020;34:3073-7. [Crossref] [PubMed]
- Alomari M, Garg P, Yazji JH, et al. Is the Organ Care System (OCS) Still the First Choice With Emerging New Strategies for Donation After Circulatory Death (DCD) in Heart Transplant? Cureus 2022;14:e26281. [Crossref] [PubMed]
- Gao L, Doyle A, Villanueva J, et al. Enhanced functional recovery of the heart donated after circulatory death determination with antemortem heparin. J Heart Lung Transplant 2020;39:607-9. [Crossref] [PubMed]
- Joshi Y, Scheuer S, Chew H, et al. Heart Transplantation From DCD Donors in Australia: Lessons Learned From the First 74 Cases. Transplantation 2023;107:361-71. [Crossref] [PubMed]
- Heylen L, Jochmans I, Samuel U, et al. The duration of asystolic ischemia determines the risk of graft failure after circulatory-dead donor kidney transplantation: A Eurotransplant cohort study. Am J Transplant 2018;18:881-9. [Crossref] [PubMed]
- Smith DE, Kon ZN, Carillo JA, et al. Early experience with donation after circulatory death heart transplantation using normothermic regional perfusion in the United States. J Thorac Cardiovasc Surg 2022;164:557-568.e1. [Crossref] [PubMed]
- Vandendriessche K, Tchana-Sato V, Ledoux D, et al. Transplantation of donor hearts after circulatory death using normothermic regional perfusion and cold storage preservation. Eur J Cardiothorac Surg 2021;60:813-9. [Crossref] [PubMed]