Effective orifice diameter: a new sizing parameter of surgical valve prostheses to inform valve selection
Introduction
Historically, the size of a surgical valve prosthesis is designated by an odd number equivalent to the annular diameter in millimeters of the smallest native valve that the prosthetic valve is intended to replace. This convention was first adopted by the valve manufacturers in the late 1970s (1) and was later codified in the international standard for cardiac valve prosthesis, ISO 5840. The actual term used to designate the valve prosthesis size evolved over the years in the standard, from the original “mounting diameter (also known as “external annulus diameter”; namely, “the diameter of the prosthetic valve where it is intended to mate with the smallest diameter of host tissue”) (2), to the later “tissue annulus diameter” (TAD; namely, “diameter in millimeters of the smallest flow area within the patient’s valve annulus”) (3). This evolution in nomenclature was due to the fact that how a prosthetic valve mates with the native valve annulus could change with intra- or supra-annular placement of the prosthetic valve (3).
The native annular diameter is typically measured intraoperatively using the largest diameter cylindrical sizer provided by the prosthetic valve manufacturer that can comfortably fit in the native annulus. This is then used to choose the size of the prosthetic valve for the patient. Perhaps because of how the prosthetic valve size is chosen (from measuring the size of the native valve orifice), it is often perceived to represent the size of the prosthetic valve orifice. In reality, however, the labeled prosthetic valve size is only a size designation (in the way that size “A”, “B”, or “C” designation would be) and may, or may not, correspond to an actual dimension on the prosthetic valve (4). Many articles have pointed out the “discrepancies” between the manufacturer-labeled prosthetic valve sizes and the measured prosthetic valve orifice sizes (5-8), with the former being one to several millimeters larger than the latter.
The discordance between the labeled prosthetic valve sizes and the actual prosthetic valve orifice sizes and the perceived equivalence between them by surgeons could have clinical implications, especially for patients with a small annulus, because the perception could give surgeons a false sense that the orifice size of a chosen prosthetic valve is large enough for a patient, while in reality it is not, which could be a potential contributing factor to prosthesis-patient mismatch. Studies have reported severe mismatch in 2–11% of patients following surgical aortic valve replacement (9) and in 5–10% of patients following surgical mitral valve replacement (10).
Ideally, the flow passage of a chosen prosthetic valve for a particular patient should match that of the native valve it replaces as closely as possible to minimize the risk of prosthesis-patient mismatch. Thus, from a hemodynamic perspective, the orifice size of a prosthetic valve is a key parameter of interest to surgeons since it is an indicator of the size of the blood flow passage through the valve. In order to increase transparency and better inform surgeons about the orifice sizes of prosthetic heart valves, the International Organization for Standardization (ISO) Cardiac Valves Working Group (the Working Group), which is responsible for developing and maintaining international standards for cardiac valve repair (11) and replacement devices (12-14), recommended in the ISO 5840-2:2021 standard, as clarified in ISO Publicly Available Specification (PAS) 7020:2023 (15), that two parameters related to prosthetic valve orifice size be provided by the manufacturers in device labeling, namely, the inflow orifice diameter and the effective orifice diameter. These, respectively, provide an upstream and downstream indicator of the orifice size of a prosthetic valve.
In order to provide repeatable and reproducible values of the inflow orifice diameter and the effective orifice diameter of a prosthetic heart valve, any methods used to obtain them need to be validated. Because the inflow orifice diameter can be physically measured and the validation of a measuring method is relatively straightforward, the Working Group decided to leave the validation of any specific measuring method to the valve manufacturer that uses it. On the other hand, there was not a published, validated method for obtaining the effective orifice diameter. Thus, the Working Group embarked on an effort to develop a validated method. Since the effective orifice diameter is calculated from the effective orifice area (EOA), the key was first to obtain the EOA in a repeatable and reproducible manner. In clinical echocardiography, the EOA of a valve is derived from the velocity of blood flow passing through the valve (16), while in benchtop hydrodynamic performance testing, it is derived from the flow rate and transvalvular pressure (12). Echocardiographic EOA measurement was ruled out because for a given valve model, the results may vary from patient to patient, as well as from time to time at the same laboratory and from laboratory to laboratory for the same patient. In addition, the echocardiographic EOA of a prosthetic heart valve newly introduced for clinical use can only be obtained after the valve has been implanted in patients, making it impossible for the manufacturer to provide the effective orifice diameter in the labeling before releasing the valve for clinical use.
EOA measurement in a pulse duplicator was also ruled out based on the finding from a prior international round-robin study carried out by the Working Group, which showed significant variabilities across participating laboratories in EOAs of the same mechanical heart valves tested under the same prescribed hemodynamic conditions (17). These led the Working Group to focus on EOA measurement under steady flow conditions. It was unknown whether EOAs measured under steady flow conditions would have less variability. Thus, the Working Group conducted another round-robin study to answer this question and determine whether the effective orifice diameter could be derived from the EOA measured under steady flow conditions in a repeatable and reproducible manner. This paper reports the results of the steady flow round-robin study.
Methods
Steady flow testing is a standardized test commonly used to characterize the hydrodynamic performance of prosthetic heart valves. It includes steady forward-flow testing, which characterizes pressure gradient across an opened prosthetic valve, and steady backflow testing, which characterizes leakage through a closed prosthetic valve. Our round-robin study focused on steady forward-flow testing. The diagram of a generic steady forward-flow tester is shown in Figure 1. During testing, a test fluid (typically a saline solution) is driven by a flow pump at a constant rate through an opened test valve mounted in a straight tube with an internal diameter of 35 mm. The pressure gradient across the test valve is measured. Four laboratories participated in the round-robin study, including Edwards Lifesciences, Irvine, CA, USA; BDC Laboratories, Wheat Ridge, CO, USA (on behalf of Medtronic, Mounds View, MN, USA, due to Medtronic resource limitations at the time of the testing activities); Abbott Medical, St. Paul, MN, USA; and Corcym, Saluggia, Vercelli, Italy. Each laboratory used their own steady flow tester. It was agreed that the test results would be anonymized. The prosthetic valves tested are listed in Table 1, which were donated by their respective manufacturers. Valves could be either the aortic or mitral model. A single set of test valves was circulated among the participating laboratories to control for valve-to-valve variability.
Table 1
Manufacturer | Valve model† |
---|---|
Mechanical heart valves | |
Abbott | Masters series (19, 25, and 31‡ mm) |
Biological heart valves | |
Abbott Medical | Epic (21, 25, and 29‡ mm) |
Trifecta (19, 21, and 25 mm) | |
Corcym | MitroFlow (23, 27, and 29 mm) |
Edwards | Magna Ease (19, 23, and 29 mm) |
Theon (31‡ mm) | |
Medtronic | Avalus (21, 25, and 27 mm) |
Mosaic (19, 23, 27‡, and 33‡ mm) |
†, one sample of each valve model and size was tested; ‡, mitral model.
In order to reflect the real-world practice of each laboratory—which could be a contributing factor to the variability in test results—each laboratory was instructed to follow Annex I, guidelines for hydrodynamic performance characterization by steady flow testing, of ISO 5840-1:2021 in performing the tests. No additional instructions were provided except that the hinges of mechanical heart valves should be oriented at 12 o’clock and 6 o’clock positions if the test tube is positioned horizontally. The study consisted of two steps: tester characterization using a standard nozzle and steady forward-flow testing of the test valves. In accordance with the standard, the tests were run over a flow rate range of 5 to 30 L/min in 5 L/min increments.
The EOA, , of a prosthetic valve is calculated using the Gorlin formula as follows (15):
where Q is the steady forward-flow rate in mL/s, is the pressure gradient in mmHg, is the density of the test fluid in g/cm3, and 51.6 is a coefficient that accounts for all required unit conversions to yield an EOA in cm2.
The effective orifice diameter, , is calculated using the formula for the area of a circle as follows:
Results
The standard nozzle-generated pressure gradient-flow rate characterization curves for the four steady forward-flow testers are shown in Figure 2, all of which agreed well with the expected curve provided in ISO 5840-1:2012 (12). The steady flow EOAs measured under various flow rates are shown in Figure 3 for representative prosthetic heart valves, which reflect a variety of design characteristics, including mechanical and biological valves, porcine (xenograft) and bovine (pericardium) tissue valves, as well as internally and externally mounted biological leaflets. Despite the differences in design characteristics among the test valves and the steady flow testers, a trend emerged from the steady flow EOA results, that is, the steady flow EOA measurement of a given valve is reproducible from laboratory to laboratory at flow rates of ≥25 L/min. Thus, the Working Group recommended that the EOA obtained at a flow rate of 25 L/min be used to derive the effective orifice diameter of a prosthetic heart valve. Table 2 summarizes the effective orifice diameter results for all the prosthetic heart valves tested. For any given valve model tested, the standard deviation of the effective orifice diameter derived experimentally was ≤0.4 mm among the four participating laboratories, indicating good inter-laboratory reproducibility of the test results.
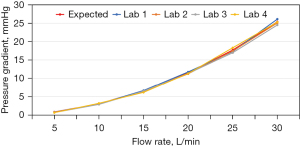
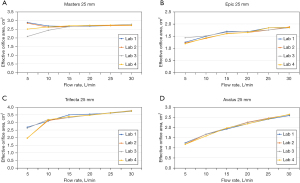
Table 2
Valve model | Effective orifice diameter at 25 L/min (mm)† | Mean ± SD (mm) | |||
---|---|---|---|---|---|
Laboratory 1 | Laboratory 2 | Laboratory 3 | Laboratory 4 | ||
Mechanical heart valves | |||||
Masters | |||||
19 mm | 12.9 | 12.8 | 12.8 | 12.9 | 12.8±0.0 |
25 mm | 18.6 | 18.5 | 18.7 | 18.7 | 18.6±0.1 |
31 mm | 26.6 | 26.7 | 26.2 | 27.1 | 26.7±0.4 |
Biological heart valves | |||||
Epic | |||||
21 mm | 11.4 | 11.6 | 11.5 | 11.5 | 11.5±0.1 |
25 mm | 15.3 | 14.9 | 14.9 | 15.3 | 15.1±0.2 |
29 mm | 17.0 | 17.0 | 17.1 | 16.9 | 17.0±0.1 |
Trifecta | |||||
19 mm | 16.0 | 16.0 | 16.1 | 15.9 | 16.0±0.1 |
21 mm | 17.7 | 17.4 | 17.5 | 17.4 | 17.5±0.1 |
25 mm | 21.4 | 21.5 | 21.5 | 21.5 | 21.5±0.0 |
MitroFlow | |||||
23 mm | 15.0 | 15.3 | 14.9 | 15.3 | 15.1±0.2 |
27 mm | 18.1 | 18.4 | 18.6 | 18.6 | 18.4±0.2 |
29 mm | 21.0 | 20.8 | 21.0 | 20.9 | 20.9±0.1 |
Magna Ease/Theon | |||||
19 mm | 14.8 | 14.8 | 15.0 | 15.1 | 14.9±0.1 |
23 mm | 16.7 | 17.1 | 17.2 | 16.8 | 17.0±0.2 |
29 mm | 22.9 | 22.9 | 23.4 | 23.0 | 23.1±0.3 |
31 mm | 23.5 | 24.4 | 24.3 | 23.8 | 24.0±0.4 |
Avalus | |||||
21 mm | 15.3 | 15.4 | 15.5 | 15.3 | 15.4±0.1 |
25 mm | 17.5 | 17.7 | 17.6 | 17.6 | 17.6±0.1 |
27 mm | 20.5 | 21.1 | 21.2 | 20.7 | 20.9±0.3 |
Mosaic | |||||
19 mm | 12.4 | 12.5 | 12.5 | 12.6 | 12.5±0.1 |
23 mm | 16.1 | 16.2 | 16.3 | 16.2 | 16.2±0.1 |
27 mm | 18.5 | 18.5 | 18.6 | 18.5 | 18.5±0.1 |
33 mm | 25.3 | 25.2 | 25.0 | 24.8 | 25.1±0.2 |
†, the effective orifice diameter values were based on a single sample for each valve model and size. Due to valve-to-valve variability, the final effective orifice diameter values reported by each manufacturer in the labeling may be different from these values. SD, standard deviation.
In order to assess intra-laboratory repeatability of the steady flow EOA results, two laboratories (Laboratory 1 and Laboratory 4) performed two measurements on the mechanical heart valve samples at flow rates of 15 and 20 L/min each. The test valves were unmounted from and then remounted into the tester between the first test runs and the repeat test runs to maximize potential intra-laboratory variability. The EOA results from this intra-laboratory repeatability study are shown in Figure 4, which shows general agreements between the first test run and second test run results, indicating good intra-laboratory repeatability of the test results.
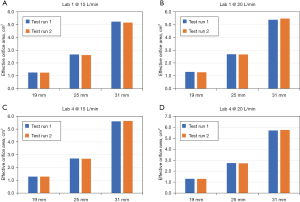
Discussion
Although both are an indicator of the flow passage of a prosthetic heart valve, there are distinctions between the effective orifice diameter introduced herein and the inflow orifice diameter more commonly used in literature (7). The inflow orifice diameter (also known as inflow internal orifice diameter) is a physical dimension, i.e., one that can be measured using a measuring tool, such as a conical gauge, or optical methods. For biological surgical valves, it is measured at the valve inflow internal orifice. Parameters varyingly related to inflow orifice diameter already exist in biological surgical valve labeling. Some example nomenclatures seen in the Instructions for Use include “internal diameter”, “stent internal diameter”, “internal orifice diameter”, and “inside diameter”. With a uniform definition of inflow orifice diameter introduced in ISO/PAS 7020:2023 (15), the valve manufacturers will start to adopt the same definition and provide the same parameter in device labeling. One limitation of the inflow orifice diameter as an indicator of the flow passage of a prosthetic valve is that it does not account for how valve leaflet (or occluder) design and opening kinematics could impact flow. As a result, two different valve models having the same inflow orifice diameter may not have the same flow passing capacity under the same hemodynamic conditions.
In contrast, the effective orifice diameter is a virtual dimension calculated from the EOA. It reflects both the inflow orifice diameter as well as the leaflet design and opening kinematics of a prosthetic heart valve. Thus, it is an indicator of the “net” flow-passing capacity of a valve. For two valves with the same labeled valve size, the one with a larger effective orifice diameter will allow more blood flow to pass through than the one with a smaller effective orifice diameter under identical hemodynamic conditions. In our study, the experimentally derived effective orifice diameters of the valves tested were found to be 3–12 mm smaller than their respective labeled valve size, confirming that the labeled size of a surgical heart valve prosthesis should not be relied upon as a sole factor for choosing a valve for a given patient. Equally, the effective orifice diameter cannot be used as a sole factor for valve selection either because there are other factors that a surgeon needs to also consider, such as anatomic fit and valve durability.
The EOA of a biological prosthetic valve exhibits a general upward trend as the flow rate increases (Figure 3), as a result of the valve leaflets opening more under a higher flow rate. The Working Group chose to use the EOA measured at a flow rate of 25 L/min to derive the effective orifice diameter due to the inter-laboratory reproducibility in EOA results that started to emerge at this flow rate. This flow rate is much higher than normal cardiac output at rest for most patients. Thus, the effective orifice diameter of a prosthetic valve derived under this flow rate would be close to the maximum effective orifice diameter that the valve could achieve. Under normal cardiac output, the effective orifice diameter would be smaller.
The Working Group set out to explore whether steady forward-flow testing is a repeatable and reproducible method for deriving the effective orifice diameters of surgical heart valve prostheses. The round-robin study demonstrated that the method is both repeatable and reproducible and is thus a valid basis for determining the effective orifice diameter. Since the main purpose of the round-robin study was not to determine the actual effective orifice diameters of the valve models tested but to develop a valid methodology for determining the effective orifice diameters, one limitation of the study was that only a single set of valves were tested. While this controlled valve-to-valve variability, it prevents the study from being a definitive determination of the final effective orifice diameters that will appear on the unit container boxes of the valves tested. Manufacturers will need to complete testing in accordance with ISO PAS 7020:2023, which includes requirements for the minimum sample size, the use of a smaller diameter tube for smaller valves, and rules for rounding results to the nearest half-millimeter (15).
Conclusions
There has long been a controversy about the labeled sizes of surgical heart valve prostheses, which is considered a contributing factor to prosthesis-patient mismatch following surgical valve replacement. The effective orifice diameter determined using a validated steady forward-flow test method provides a repeatable and reproducible characterization of the hemodynamic characteristics of a surgical valve prosthesis. As part of the ISO 5840-2:2021 standard, as clarified in ISO PAS 7020:2023, this new sizing parameter, along with other key parameters, will soon be adopted by all surgical valve manufacturers and printed on the valve unit container box to inform valve selection by surgeons.
Acknowledgments
We thank Edwards Lifesciences, Medtronic, Abbott Medical, and Corcym for providing the test valves.
Funding: None.
Footnote
Conflicts of Interest: The authors have no conflicts of interest to declare.
Disclaimer: The findings and conclusions in this article have not been formally disseminated by the U.S. FDA and should not be construed to represent any agency determination or policy. The mention of commercial products, their sources, or their use in connection with material reported herein is not to be construed as either an actual or implied endorsement of such products by the U.S. Department of Health and Human Services.
Open Access Statement: This is an Open Access article distributed in accordance with the Creative Commons Attribution-NonCommercial-NoDerivs 4.0 International License (CC BY-NC-ND 4.0), which permits the non-commercial replication and distribution of the article with the strict proviso that no changes or edits are made and the original work is properly cited (including links to both the formal publication through the relevant DOI and the license). See: https://creativecommons.org/licenses/by-nc-nd/4.0/.
References
- Diggelmann UP, Krayenbühl HP, Rothlin M, et al. The Bjoerk-Shiley and the Lillehei-Kaster valve in aortal position. A hemodynamic comparison. Schweiz Med Wochenschr 1978;108:92-7.
- International Organization for Standardization. ISO 5840:1984. Implants for surgery - Cardiovascular implants - Cardiac valve prostheses. Geneva: ISO; 1984.
- International Organization for Standardization. ISO 5840:2005. Cardiovascular implants - Cardiac valve prostheses. Geneva: ISO; 2005.
- Chambers JB, Oo L, Narracott A, et al. Nominal size in six bileaflet mechanical aortic valves: a comparison of orifice size and biologic equivalence. J Thorac Cardiovasc Surg 2003;125:1388-93. [Crossref] [PubMed]
- Cochran RP, Kunzelman KS. Discrepancies between labeled and actual dimensions of prosthetic valves and sizers. J Card Surg 1996;11:318-24; discussion 325. [Crossref] [PubMed]
- Christakis GT, Buth KJ, Goldman BS, et al. Inaccurate and misleading valve sizing: a proposed standard for valve size nomenclature. Ann Thorac Surg 1998;66:1198-203. [Crossref] [PubMed]
- van Boxtel AGM, Mariani MA, Ebels T. All surgical supra-annular aortic valvar tissue prostheses are labelled too large. Interdiscip Cardiovasc Thorac Surg 2023;36:ivad076. [Crossref] [PubMed]
- Yang B, Ghita C, Makkinejad A, et al. Early outcomes of the Y-incision technique to enlarge the aortic annulus 3 to 4 valve sizes. J Thorac Cardiovasc Surg 2024;167:1196-1205.e2. [Crossref] [PubMed]
- Pibarot P, Dumesnil JG. Prosthesis-patient mismatch: definition, clinical impact, and prevention. Heart 2006;92:1022-9. [Crossref] [PubMed]
- Pibarot P, Dumesnil JG. Prosthesis-patient mismatch in the mitral position: old concept, new evidences. J Thorac Cardiovasc Surg 2007;133:1405-8. [Crossref] [PubMed]
- International Organization for Standardization. ISO 5910:2018. Cardiovascular implants and extracorporeal systems - Cardiac valve repair devices. Geneva: ISO; 2018.
- International Organization for Standardization. ISO 5840-1:2021. Cardiovascular implants - Cardiac valve prostheses - Part 1: General requirements. Geneva: ISO; 2021.
- International Organization for Standardization. ISO 5840-2:2021. Cardiovascular implants - Cardiac valve prostheses - Part 2: Surgically implanted heart valve substitutes. Geneva: ISO; 2021.
- International Organization for Standardization. ISO 5840-3:2021. Cardiovascular implants - Cardiac valve prostheses - Part 3: Heart valve substitutes implanted by transcatheter techniques. Geneva: ISO; 2021.
- International Organization for Standardization. ISO/PAS 7020:2023. Sizing parameters of surgical valve prostheses: Requirements regarding the application of ISO 5840-2. Geneva: ISO; 2023.
- Garcia D, Kadem L. What do you mean by aortic valve area: geometric orifice area, effective orifice area, or gorlin area? J Heart Valve Dis 2006;15:601-8.
- Wu C, Saikrishnan N, Chalekian AJ, et al. In-Vitro Pulsatile Flow Testing of Prosthetic Heart Valves: A Round-Robin Study by the ISO Cardiac Valves Working Group. Cardiovasc Eng Technol 2019;10:397-422. [Crossref] [PubMed]