Current approaches to spinal cord protection during open thoracoabdominal aortic aneurysm repair
Introduction
Spinal cord deficit (SCD) is the most feared complication after open descending thoracic and thoracoabdominal aortic aneurysm (TAAA) repair and is associated with increased morbidity and mortality. SCD is defined as the development of postoperative paraplegia, paraparesis, or bladder/bowel dysfunction after repair. This can occur either during surgery and manifest when the patient is awake, or postoperatively after the patient initially appears to be neurologically intact. In 1986, Crawford et al. reported that the incidence of SCD in patients who underwent extent II TAAA repair was 28% (1). In 2019, after continued technique refinements and advances, the rate of permanent SCD in patients who underwent extent II TAAA repair was 8% and approached 5% for all extents at an experienced center (2) (Figure 1). A large meta-analysis of 169 studies and 22,634 patients demonstrated that the overall incidence of SCD for open TAAA repairs was 7.0% and that the extent of repair remained a primary determinant of SCD risk (extent I, 4%; extent II, 15%; extent III, 7%; extent IV, 2%; and extent V, 7%) (3). The focus of this discussion will be on operative techniques and the perioperative approach to reduce the risk of SCDs.
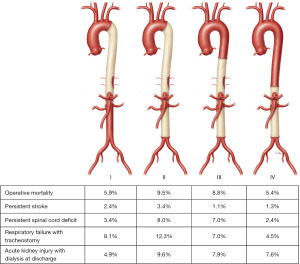
Fundamentals of operative management
Established risk factors for perioperative SCD include a variety of preoperative features, clinical presentations, and anatomical and intraoperative factors that can increase SCD risk, especially when multiple components are present. Other risk predictors are the presence of chronic symptoms, an acute aortic dissection/rupture, extensive thoracoabdominal aortic disease, and intraoperative hypotension (Table 1). In general, patients at a high risk of SCD are of advanced age and typically do not have Marfan syndrome or other heritable thoracic aortic diseases. The initial preoperative evaluation focuses on a broad review of the patient, including comorbidities (4). Those that are modifiable are targeted for preoperative intervention; however, in our experience, at least 20% of patients present with an urgent or emergency need for intervention, thereby limiting that opportunity.
Table 1
Preoperative |
Advanced age |
Previous abdominal aortic surgery |
Chronic kidney disease |
Presentation |
Emergency surgery |
Aortic dissection/rupture |
Intraoperative |
Level of aortic cross clamping; greater anatomic extent of TAAA repair |
Exclusion of the hypogastric artery |
Failure to reattach critical intercostal arteries |
Left subclavian artery exclusion |
Degree of hypothermia |
Period of distal ischemia |
TAAA, thoracoabdominal aortic aneurysm.
Historically, “clamp and sew” was a common technique for TAAA repair. Throughout the last three decades, a variety of techniques have been incorporated into a multimodal approach that we currently use for all TAAA extents. With an emphasis on an expeditious repair, this approach involves the use of mild passive hypothermia (32–34 ℃, nasopharyngeal), left heart bypass (LHB), moderate heparinization (1 mg/kg), sequential aortic clamping with distal aortic perfusion whenever feasible, and judicious reattachment of intercostal and lumbar arteries (RILA) (5). Proximal aortic cross-clamping results in increased central venous and intrathecal cerebral spinal fluid (CSF) pressure with an inverse relationship to spinal cord perfusion. Ischemic spinal cord neurons release free radicals and other excitatory neurotransmitters with deleterious consequences exacerbated by reperfusion injury (6). Visceral protection, when feasible, can include cold crystalloid or crystalloid cardioplegic (off-label use of Custodiol-HTK; Essential Pharmaceuticals, Ewing Township, NJ) perfusion for renal protection, and isothermic blood perfusion for mesenteric vessels (7). Although others advocate for the routine use of deep hypothermic circulatory arrest (8), we reserve this approach for a small number of cases (3% in extent I; <2% in extent II repairs) where the distal arch/proximal descending thoracic aorta cannot be clamped (2).
Below, we highlight important operative steps during open descending thoracic and TAAA repair and discuss preventive strategies to reduce the risk of SCD.
Cerebrospinal fluid drainage (CSFD)
CSFD is a vital component of spinal cord protection that works by lowering intrathecal CSF pressure. Our group has shown in a randomized trial that the use of CSFD in extent I and II TAAA repairs resulted in an 80% relative risk reduction [absolute risk reduction, 10.4%; number needed to treat (NNT), 9.6 patients] in the development of SCD (9). On the basis of that trial and two other randomized trials (10), CSFD is considered a Class I (level of evidence A) recommendation in the 2022 American College of Cardiology/American Heart Association guidelines (11) and a Class I (level of evidence B) recommendation in the 2014 European Society of Cardiology guidelines (12). Currently, our CSFD use rates are as follows: extent I, 97%; extent II, 98%; extent III, 77%; extent IV, 36% (2,13).
At the start of the procedure, the CSFD is placed by an experienced anesthesiologist by inserting a 14-G needle into the lower lumbar interspaces (L3–4, L4–5) until CSF is reached. If blood is encountered during lumbar puncture before an elective case, the procedure is postponed, or the CSFD catheter is placed at the end of the procedure (Video). In cases of hemodynamic instability from an acute presentation, the CSF drain is not placed at the start of the procedure because expeditious aortic control is necessary if rupture is suspected. At the conclusion of the procedure, the patient is rolled from the supine position to the right decubitus position, and a catheter is placed before the patient is transferred from the operating room to the intensive care unit. In difficult cases, interventional radiology consultation is needed for CSF drain placement under fluoroscopy either before or after TAAA repair (14).
In adults, CSF pressure typically fluctuates between 8 and 24 mmHg and varies with position. For example, CSF pressure is lower when the patient is in the lateral decubitus position (8–15 mmHg) than when the patient is sitting up (16–24 mmHg). CSF pressure has also been found to increase with body mass index and decrease with advanced age (15,16).
In our practice, CSF is passively drained to maintain a pressure <15 mmHg during aortic clamping. Although there is not a hard cutoff, we typically do not drain more than 30 to 40 mL/hour during surgery, except under rare circumstances. During the early postoperative recovery period, we aim for a goal of 15 to 18 mmHg CSF pressure by draining up to 10 mL/hour, with a maximum of 25 mL in 4 hours or 150 mL in 24 hours (Table 2) (17).
Table 2
Category | Specific strategy | Comments |
---|---|---|
Intercostal arteries | ||
Direct reattachment | Infrequent risk of late patch aneurysm, especially in patients with heritable thoracic aortic disease | |
Preconditioning | Coiling, PAPA-ARTIS trial (17) | |
Monitoring | ||
Motor evoked potentials | Risk of false positives | |
Complexity of anesthesia management | ||
Somatosensory evoked potentials | Mixed results | |
CSF drainage | ||
Prophylactic | Standard of care. Near universal for extent I and II TAAA repairs and most extent III repairs. Used selectively in extent IV repairs, typically those with prior abdominal aortic repair | |
Rescue | Vigilant postoperative management. Early recognition of spinal cord deficit treated with higher mean arterial pressure, CSF drainage, steroids, and mannitol | |
Distal perfusion | ||
Left heart bypass | Most commonly utilized in extent I and II repairs | |
Cardiopulmonary bypass ± hypothermic circulatory arrest | Selectively used | |
Pharmacology | Predominantly animal models; little validated human clinical data | |
Papaverine | Dilates spinal cord vessels | |
Mannitol | Reduces intracellular metabolism, free radical scavenger | |
Barbiturates | Reduces spinal cord oxygen demand | |
Steroids | Reduces inflammation and stabilizes membranes | |
Naloxone | Blocks excitatory neurotransmitters | |
Hypothermia | ||
Passive cooling | Some evidence to suggest protection | |
Regional cooling | Some evidence to suggest protection | |
Systemic hypothermic circulatory arrest | Selectively used |
CSF, cerebral spinal fluid; TAAA, thoracoabdominal aortic aneurysm.
In general, after a 6- to 12-hour test clamp trial, the CSFD catheter is removed after 48 to 72 hours, but the drain may remain longer if there are neurologic concerns or if there has been a high CSF pressure with significant drainage.
Blood pressure management
A critical element of preventing SCD is maintaining an adequate spinal cord perfusion pressure (SCPP), which is equal to the spinal arterial pressure minus the venous pressure. Using values that are more easily measured, this can be estimated as the mean arterial pressure (MAP) minus the CSF pressure. The goal is to maintain an SCPP that is at least 60 mmHg (e.g., commonly achieved with a MAP of 80 mmHg and a CSF pressure of 15 mmHg), because values below that are associated with a higher SCD risk (18).
Etz and Griepp developed the concept of the collateral network by demonstrating that SCPP dropped to 50% to 75% of the systemic MAP according to the number of segmental arteries ligated in pigs or humans (19). This in turn reinforced the practice of aiming for a higher systemic MAP to provide a margin for maintaining adequate SCPP.
Patient positioning
For CSF drain placement, the patient is positioned on a beanbag and into a right lateral decubitus position. Next, the patient’s shoulders are turned to a 60-degree angle and the hips are flexed to 30 degrees. The chest is typically entered in the 5th intercostal space or lower, depending on the extent of repair.
Left heart bypass
The role of LHB was established in a retrospective study that showed an associated 63% relative risk reduction in SCD for patients undergoing extent II repair (absolute risk reduction, 8.5%, P=0.007; NNT =12) (20). In a recent series of patients from our center, LHB was used in 45% of TAAA repairs with a mean duration of 25 minutes; LHB was used in 61% of extent I, 82% of extent II, 7% of extent III, and 1% of extent IV repairs (2).
Reattachment of intercostal and lumbar arteries
Typically, the intercostal and lumbar arteries are aggressively reimplanted in the T7/8 to L1/2 region and revascularized. Commonly used techniques include a patch or a small graft (21). The tradeoff is that another graft increases the complexity of the repair, whereas preserving the native patch of aorta to reattach intercostals increases the risk of late complications (e.g., patch aneurysm) from the reattachment, especially in patients with Marfan syndrome or other heritable thoracic conditions (Figure 2). Selective intercostal or lumbar arteries are preferably contiguous and include 2 to 3 pairs of arteries without evidence of notable back bleeding. RILA is used in 51% of TAAA repairs, with the following breakdown by extent: extent I, 55%; extent II, 88%; extent III, 34%; and extent IV, 2% (2). RILA has been shown to be an independent predictor of reduced persistent (relative risk ratio, 0.36) and delayed (relative risk ratio, 0.49) SCD, cutting the SCD risk by about half (22). Widespread variation in techniques ranging from selective reimplantation based on intraoperative neuromonitoring to routine implantation based on patency and anatomic location has not been associated with any major differences in SCD, suggesting that the role of RILA remains incompletely understood. Although case reports of endovascular preconditioning by embolization before definitive repair have demonstrated efficacy, further studies of appropriate patient selection and the role for this strategy are needed (23).
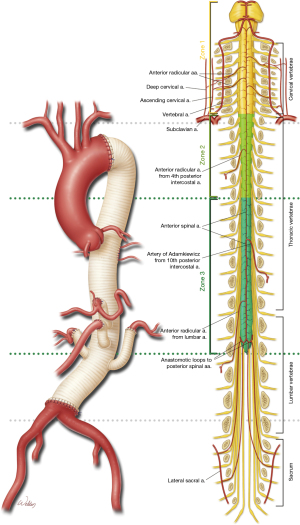
Intraoperative monitoring techniques
In some cases, intraoperative or even postoperative spinal cord neuromonitoring has been performed, although its widespread clinical use has been limited (Table 2) (17). This is most likely because of non-expert implementation, ambiguity in interpretation, and mixed outcomes in reporting.
Motor evoked potential (MEP)
To measure MEP, a signal is sent from the cranium to the anterior horn motor neuron to produce a leg muscle twitch. The amplitude of the signal is then measured as it travels down the spinal cord. A loss of signal indicates at least a temporary fall in SCPP and the possible loss of a crucial intercostal artery (ICA). This can be mitigated by reattaching the corresponding ICA. A systematic review of 19 studies of varying degrees of quality showed that MEP has an overall sensitivity rate of 89% and specificity of 95% for detecting intraoperative or postoperative SCD (24). Although heterogeneity was observed with respect to cutoff points across studies, an all-or-none approach generally appeared to be a better predictor of SCD.
Notably, the anesthetic regimen must be modified when using MEP. After an initial dose of paralytic on induction for intubation, paralytics are not administered again until the patient is ready for closure. Inhalation anesthetic agents and nitrous oxide may impair the residual MEP signal and must be accounted for with this strategy.
Somatosensory evoked potential (SSEP)
To measure SSEP, a signal is sent from the tibial nerve to the cranium (cortex). A stimulus is sent every 3 seconds, alternating between both sides of the body, and the average amplitudes of the signal are measured. SSEP tests the functionality of the dorsal column (white matter/axons) by using diminished SSEP signals as a guide for RILA or as a predictor of SCD (25,26). In general, the MEP is considered a more sensitive technique, whereas the SSEP is considered a more specific measure for assessing spinal cord function (27).
Others have also advocated for the use of direct epidural cold perfusate infusion for regional spinal hypothermia (10–12 ℃), the use of naloxone or barbiturate derivatives to reduce spinal metabolism, or intrathecal papaverine (28). Many of these techniques are under investigation.
Fundamentals of postoperative management
Postoperative management after TAAA repair must complement the operative procedure. General guiding principles include maintaining a satisfactory MAP (i.e., 80–100 mmHg) with adequate volume resuscitation and cardiac index, CSFD management, optimal hemoglobin thresholds (i.e., 10 g/dL), adequate oxygenation in the early postoperative period to ensure adequate spinal cord oxygen delivery, and careful multidisciplinary critical care (29,30). Complications from CSFD can occur in up to 10% of cases, with headache being most common. In those patients, close vigilance and care protocols can facilitate early recognition and timely management (31). In up to 3% of cases, intracerebral hemorrhage may develop (most commonly subdural hematoma, 2%). Severe headache with nausea, vomiting, or focal neurologic deficits warrants immediate computed tomographic imaging and possible neurosurgical intervention (30).
Management of delayed paraplegia
Delayed paraplegia is defined as SCD that develops later in the postoperative period after the patient has awakened from anesthesia appearing neurologically intact after TAAA repair. Cases of delayed paraplegia are often preceded by periods of hypotension that may be triggered by arrhythmias, hypovolemia, or bleeding that underscore the importance of closely monitoring blood pressure throughout a patient’s hospital course (32,33).
In patients with delayed paraplegia, a mean arterial blood pressure of >100 mmHg, a CSF pressure closer to 10 mmHg, and adequate volume and hemoglobin levels are desirable. We allow CSF drainage up to 40 mL every 4 hours, and the use of steroids and mannitol are initiated (consistent with the previously described COPS protocol or CSF drain status, Oxygen delivery, and Patient Status algorithm) (30,34). Our current approach to delayed paraplegia is outlined in Figure 3 (30).
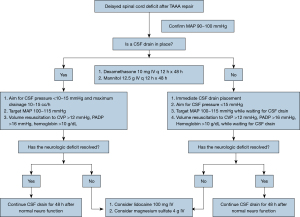
Conclusions
TAAA repair is associated with a significant risk of SCD. Over the course of several decades, multiple surgical adjuncts and strategies have been developed that have reduced the risk of SCD substantially when performed by experienced surgeons and teams. To further reduce the risk of SCD in patients who undergo TAAA repair, continued research is necessary.
Acknowledgments
We appreciate the editorial support of Susan M. Green, MPH and Nicole Stancel, PhD, ELS(D), of The Texas Heart Institute’s Scientific Publications Department. We also appreciate the graphic artwork of Scott Weldon, MA, CMI, FAMI, of the Division of Cardiothoracic Surgery at Baylor College of Medicine.
Funding: None.
Footnote
Conflicts of Interest: JSC participates in clinical studies with and/or consults for Terumo Aortic, Medtronic, W. L. Gore & Associates, CytoSorbents, Edwards Lifesciences, and Abbott Laboratories and receives royalties and grant support from Terumo Aortic. SAL consults for Terumo Aortic and Cerus and serves as a principal investigator for clinical studies sponsored by Terumo Aortic and CytoSorbents. SAL’s work is supported in part by the Jimmy and Roberta Howell Professorship in Cardiovascular Surgery at Baylor College of Medicine. OP provides consultation for and participates in clinical trials with Medtronic and W.L. Gore & Associates. MRM serves on the advisory board for Medtronic and Edwards Lifesciences. SC has served on advisory boards for Edwards Lifesciences, La Jolla Pharmaceutical Company, Eagle Pharmaceuticals and Baxter Pharmaceuticals. The other authors have no conflicts of interest to declare.
Open Access Statement: This is an Open Access article distributed in accordance with the Creative Commons Attribution-NonCommercial-NoDerivs 4.0 International License (CC BY-NC-ND 4.0), which permits the non-commercial replication and distribution of the article with the strict proviso that no changes or edits are made and the original work is properly cited (including links to both the formal publication through the relevant DOI and the license). See: https://creativecommons.org/licenses/by-nc-nd/4.0/.
References
- Crawford ES, Crawford JL, Safi HJ, et al. Thoracoabdominal aortic aneurysms: preoperative and intraoperative factors determining immediate and long-term results of operations in 605 patients. J Vasc Surg 1986;3:389-404. [Crossref] [PubMed]
- Coselli JS, LeMaire SA, Preventza O, et al. Outcomes of 3309 thoracoabdominal aortic aneurysm repairs. J Thorac Cardiovasc Surg 2016;151:1323-37. [Crossref] [PubMed]
- Gaudino M, Khan FM, Rahouma M, et al. Spinal cord injury after open and endovascular repair of descending thoracic and thoracoabdominal aortic aneurysms: A meta-analysis. J Thorac Cardiovasc Surg 2022;163:552-64. [Crossref] [PubMed]
- Chatterjee S, Casar JG, LeMaire SA, et al. Perioperative care after thoracoabdominal aortic aneurysm repair: The Baylor College of Medicine experience. Part 1: Preoperative considerations. J Thorac Cardiovasc Surg 2021;161:693-8. [Crossref] [PubMed]
- Coselli JS, de la Cruz KI, Preventza O, et al. Extent II thoracoabdominal aortic aneurysm repair: How I do it. Semin Thorac Cardiovasc Surg 2016;28:221-37. [Crossref] [PubMed]
- Wynn MM, Acher CW. A modern theory of spinal cord ischemia/injury in thoracoabdominal aortic surgery and its implications for prevention of paralysis. J Cardiothorac Vasc Anesth 2014;28:1088-99. [Crossref] [PubMed]
- Kahlberg A, Tshomba Y, Baccellieri D, et al. Renal perfusion with histidine-tryptophan-ketoglutarate compared with Ringer's solution in patients undergoing thoracoabdominal aortic open repair. J Thorac Cardiovasc Surg 2023;165:569-579.e5. [Crossref] [PubMed]
- Kouchoukos NT, Masetti P, Rokkas CK, et al. Hypothermic cardiopulmonary bypass and circulatory arrest for operations on the descending thoracic and thoracoabdominal aorta. Ann Thorac Surg 2002;74:S1885-7; discussion S1892-8. [Crossref] [PubMed]
- Coselli JS, LeMaire SA, Köksoy C, et al. Cerebrospinal fluid drainage reduces paraplegia after thoracoabdominal aortic aneurysm repair: results of a randomized clinical trial. J Vasc Surg 2002;35:631-9. [Crossref] [PubMed]
- Khan SN, Stansby G. Cerebrospinal fluid drainage for thoracic and thoracoabdominal aortic aneurysm surgery. Cochrane Database Syst Rev 2012;10:CD003635.
- Isselbacher EM, Preventza O, Hamilton Black J 3rd, et al. 2022 ACC/AHA Guideline for the Diagnosis and Management of Aortic Disease: A Report of the American Heart Association/American College of Cardiology Joint Committee on Clinical Practice Guidelines. Circulation 2022;146:e334-482. [Crossref] [PubMed]
- Erbel R, Aboyans V, Boileau C, et al. 2014 ESC Guidelines on the diagnosis and treatment of aortic diseases: Document covering acute and chronic aortic diseases of the thoracic and abdominal aorta of the adult. The Task Force for the Diagnosis and Treatment of Aortic Diseases of the European Society of Cardiology (ESC). Eur Heart J 2014;35:2873-926. [Crossref] [PubMed]
- Chatterjee S, Preventza O, Orozco-Sevilla V, et al. Critical care management after open thoracoabdominal aortic aneurysm repair. J Cardiovasc Surg (Torino) 2021;62:220-9. [Crossref] [PubMed]
- Katz A, Suero OR, Orozco-Sevilla V, et al. Optimal perioperative care for thoracoabdominal and descending thoracic aortic aneurysm repair: a review. Vessel Plus 2023;7:1.
- Ren R, Wang N, Zhang X, et al. Cerebrospinal fluid pressure correlated with body mass index. Graefes Arch Clin Exp Ophthalmol 2012;250:445-6. [Crossref] [PubMed]
- Fleischman D, Berdahl JP, Zaydlarova J, et al. Cerebrospinal fluid pressure decreases with older age. PLoS One 2012;7:e52664. [Crossref] [PubMed]
- Petroff D, Czerny M, Kölbel T, et al. Paraplegia prevention in aortic aneurysm repair by thoracoabdominal staging with 'minimally invasive staged segmental artery coil embolisation' (MIS2ACE): trial protocol for a randomised controlled multicentre trial. BMJ Open 2019;9:e025488. [Crossref] [PubMed]
- Squair JW, Bélanger LM, Tsang A, et al. Spinal cord perfusion pressure predicts neurologic recovery in acute spinal cord injury. Neurology 2017;89:1660-7. [Crossref] [PubMed]
- Etz CD, Zoli S, Bischoff MS, et al. Measuring the collateral network pressure to minimize paraplegia risk in thoracoabdominal aneurysm resection. J Thorac Cardiovasc Surg 2010;140:S125-30; discussion S142-S146. [Crossref] [PubMed]
- Coselli JS, LeMaire SA. Left heart bypass reduces paraplegia rates after thoracoabdominal aortic aneurysm repair. Ann Thorac Surg 1999;67:1931-4; discussion 1953-8. [Crossref] [PubMed]
- Woo EY, Mcgarvey M, Jackson BM, et al. Spinal cord ischemia may be reduced via a novel technique of intercostal artery revascularization during open thoracoabdominal aneurysm repair. J Vasc Surg 2007;46:421-6. [Crossref] [PubMed]
- Coselli JS, Green SY, Price MD, et al. Spinal cord deficit after 1114 extent II open thoracoabdominal aortic aneurysm repairs. J Thorac Cardiovasc Surg 2020;159:1-13. [Crossref] [PubMed]
- Etz CD, Debus ES, Mohr FW, et al. First-in-man endovascular preconditioning of the paraspinal collateral network by segmental artery coil embolization to prevent ischemic spinal cord injury. J Thorac Cardiovasc Surg 2015;149:1074-9. [Crossref] [PubMed]
- Tanaka Y, Kawaguchi M, Noguchi Y, et al. Systematic review of motor evoked potentials monitoring during thoracic and thoracoabdominal aortic aneurysm open repair surgery: a diagnostic meta-analysis. J Anesth 2016;30:1037-50. [Crossref] [PubMed]
- Achouh PE, Estrera AL, Miller CC 3rd, et al. Role of somatosensory evoked potentials in predicting outcome during thoracoabdominal aortic repair. Ann Thorac Surg 2007;84:782-7; discussion 787-8. [Crossref] [PubMed]
- Pillai JB, Pellet Y, Panagopoulos G, et al. Somatosensory-evoked potential-guided intercostal artery reimplantation in thoracoabdominal aortic aneurysm surgery. Innovations (Phila) 2013;8:302-6. [Crossref] [PubMed]
- Tanaka A, Safi HJ, Estrera AL. Current strategies of spinal cord protection during thoracoabdominal aortic surgery. Gen Thorac Cardiovasc Surg 2018;66:307-14. [Crossref] [PubMed]
- Svensson LG. An approach to spinal cord protection during descending or thoracoabdominal aortic repairs. Ann Thorac Surg 1999;67:1935-58.
- Chung JC, Lodewyks CL, Forbes TL, et al. Prevention and management of spinal cord ischemia following aortic surgery: A survey of contemporary practice. J Thorac Cardiovasc Surg 2022;163:16-23.e7. [Crossref] [PubMed]
- Chatterjee S, Casar JG, LeMaire SA, et al. Perioperative care after thoracoabdominal aortic aneurysm repair: The Baylor College of Medicine experience. Part 2: Postoperative management. J Thorac Cardiovasc Surg 2021;161:699-705. [Crossref] [PubMed]
- Youngblood SC, Tolpin DA, LeMaire SA, et al. Complications of cerebrospinal fluid drainage after thoracic aortic surgery: a review of 504 patients over 5 years. J Thorac Cardiovasc Surg 2013;146:166-71. [Crossref] [PubMed]
- Wong DR, Coselli JS, Amerman K, et al. Delayed spinal cord deficits after thoracoabdominal aortic aneurysm repair. Ann Thorac Surg 2007;83:1345-55; discussion 1355. [Crossref] [PubMed]
- Maniar HS, Sundt TM 3rd, Prasad SM, et al. Delayed paraplegia after thoracic and thoracoabdominal aneurysm repair: a continuing risk. Ann Thorac Surg 2003;75:113-9; discussions 119-20.
- Estrera AL, Sheinbaum R, Miller CC, et al. Cerebrospinal fluid drainage during thoracic aortic repair: safety and current management. Ann Thorac Surg 2009;88:9-15; discussion 15. [Crossref] [PubMed]