Left ventricular decompression in veno-arterial extracorporeal membrane oxygenation
Introduction
While veno-arterial extracorporeal membrane oxygenation (VA-ECMO) effectively restores systemic blood flow in patients with cardiogenic shock, it has potentially deleterious effects on the left ventricle (LV).
VA-ECMO increases aortic root pressure and LV afterload, which can also lead to LV distention, especially if there is any aortic valve incompetence. LV distention is exacerbated by other sources of left atrial inflow (including bronchial and Thebesian veins, and residual pulmonary blood flow) (1-3). Hence VA-ECMO can decrease LV stroke volume, and increase LV end-systolic and end-diastolic volumes (4).
Increased LV end-diastolic pressure in turn increases myocardial wall stress, which can cause ischemia and ventricular arrhythmias (4,5). Furthermore, although VA-ECMO reduces right ventricular (RV) preload (via venous drainage), RV afterload may be increased due to pulmonary venous hypertension secondary to LV-volume overload (4). This can lead to pulmonary vascular injury and hemorrhage, acute respiratory distress syndrome, and severe pulmonary edema (6).
The combination of increased LV diastolic pressure and afterload may in some patients completely inhibit aortic valve opening. Reduced or absent LV systolic ejection promotes intracardiac thrombus formation, which can cause fatal thromboembolism. LV thrombus extension into the pulmonary vein and distal pulmonary vasculature further limits the prospect of myocardial recovery and is a contraindication to long-term support with a ventricular assist device (VAD) (6,7).
Methods
Five electronic databases, including MEDLINE, EMBASE, PubMed, Cochrane Central Register of Controlled Trials (CENTRAL), and Cochrane Database of Systematic Reviews, were searched for original published studies from their dates of inception to January 2018. To maximize sensitivity of the search strategy, the following terms were used: “ecmo” or “extracorporeal membrane oxygenation” or “extracorporeal circulation” or “extracorporeal life support” AND “left ventric*” or “left heart” AND “unload*” or “decompress*” or “distension” or “distention”, as either keywords or MeSH terms. The reference lists of retrieved articles also were reviewed for additional relevant studies. Inclusion criteria included adult and pediatric studies that investigated LV overload in VA-ECMO and were published in the English language. All types of studies, including case reports and series, observational studies, review articles, and animal studies were included. Exclusion criteria included abstracts and conference presentations.
Incidence
The current data on the incidence of LV distention in adult and pediatric VA-ECMO patients is limited and highly variable (from 1% to 68%) (1,7,8). In a retrospective review of 134 adult VA-ECMO patients, Eliet et al. [2018] reported that 27 (20%) required LV unloading, while Truby et al. [2017] found that 36 of 121 adults on VA-ECMO (30%) had evidence of LV distention, including nine patients requiring immediate venting (7,9). The wide variation in reported rates of LV distention is likely due to a lack of standard diagnostic criteria for LV overload, as well as variations in study populations and ECMO practice.
Diagnosis
Currently, there are no standardized guidelines for diagnosing LV distention in VA-ECMO patients. The studies included in the present review used one or more of the criteria summarized in Table 1.
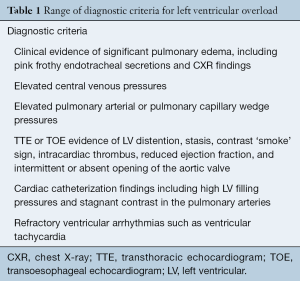
Full table
Truby and colleagues [2017] proposed diagnostic criteria for LV distention for use within the first two hours of VA-ECMO support (7). These criteria characterized patients as: ‘no LV distention (LVD)’, ‘subclinical LVD’—not requiring immediate decompression, and ‘clinical LVD’—requiring emergency decompression (7). Subclinical LVD was diagnosed from chest X-ray (CXR) evidence of pulmonary edema and a pulmonary arterial diastolic blood pressure (PADBP) greater than 25 mmHg (7). Clinical LVD was defined as severe pulmonary edema with worsening oxygenation, refractory ventricular arrhythmia, and/or significant LV stasis and increased LV end-diastolic diameter (LVEDD) on echocardiography requiring immediate LV decompression (7).
In regards to CXR evidence of pulmonary vascular injury during VA-ECMO support, Cheng et al. [2013] noted that the severity of pulmonary vascular injury may initially be under-recognized due to reduced pulmonary perfusion during VA-ECMO support, resulting in a relatively unremarkable CXR (6). However, once pulmonary blood flow is restored, radiological signs of florid pulmonary edema may become more apparent (6).
While transthoracic echocardiography (TTE) is useful in detecting LV distention in adults, diagnostic criteria are less well established in children. Eckhauser et al. [2014] demonstrated that TTE did not always provide obvious evidence of left heart hypertension in three peripheral VA-ECMO patients (aged 2 to 12 years) that were later demonstrated to have severe LV distention on cardiac catheterization (10). The echocardiographic parameters examined in this study included interatrial pressure gradient, left atrial diameter, and assessment of LV function (10). A major reason for this difficulty is that there are no widespread and standardized guidelines that specify which echocardiographic parameters are most useful in this pediatric setting. The authors in this study thereby developed a pediatric diagnostic echocardiographic algorithm derived from adult guidelines, though this clearly requires further investigation and application to larger patient sample sizes (10).
Serial brain natriuretic peptide (BNP) measurements have also been suggested as a means of monitoring LV distention and effectiveness of decompression, although this requires further investigation and validation (11).
Risk factors and prevention
The main risk factors for LV distention on VA-ECMO include:
- Severe LV failure (1,7). VA-ECMO exacerbates LV failure by increasing LV afterload, which can cause systolic closure of the aortic valve;
- Aortic insufficiency (12). Any degree of aortic sufficiency may exacerbate LV overload. While mitral regurgitation alleviates LV pressure overload, it worsens pulmonary edema (5,7,13);
- High ECMO flow rate. The increase in afterload due to VA-ECMO is directly related to pump flow rate (12);
- Intravascular volume overload due to excessive fluid administration, as it may contribute to increased LV filling pressures (1).
LV distention may therefore be managed with vasodilators, inotropes, decreasing ECMO pump flow and lowering LV preload (with hemofiltration and judicious fluid administration). The adverse pulmonary effects of VA-ECMO can be counteracted with the use of low tidal volumes, increased positive end-expiratory pressures (PEEP), and early extubation when feasible (1,12).
Indications and timing for decompression
There are no consensus guidelines as to when LV venting should be performed. Some centers perform this prophylactically and routinely, particularly for pediatric patients (1). This is because infants have lower myocardial compliance than adults, which makes them more potentially more vulnerable to LV distention and its sequelae (1). Other pediatric centers vent the LV if the PCWP is greater than 18 mmHg, even in the absence of severe pulmonary edema (14). However, as will be discussed, LV venting itself is associated with significant risks.
Conversely, in adults, although some degree of LV distention probably occurs in nearly all VA-ECMO patients, this can be quite well tolerated with medical management alone (1). Generally recognized indications for LV venting include:
- Aortic valve closure, due to the high risk of ventricular stasis and thrombosis (1);
- Severe aortic regurgitation, which will exacerbate LV overload and distention on VA-ECMO (1);
- Severe, refractory pulmonary edema secondary to LV overload (1);
- Patients who are not candidates for early left ventricular assist device (LVAD) support. Rupprecht et al. [2013] suggested that VA-ECMO with LV venting be used for patients with high recovery potential (e.g., acute myocarditis), while early LVAD implantation should be considered in patients with low recovery potential (1).
Other indications for LV decompression that have been reported include:
- Distended left atrium (LA) and LV with elevated pressures despite maximal pharmacological measures (15);
- Pulmonary hemorrhage (15);
- Evidence of elevated LV wall stress (15);
- Severe or persistent LV dysfunction (15).
Techniques for decompression
A wide variety of LV venting techniques have been reported in the literature. These can be categorized into surgical and percutaneous approaches, which are summarized in Table 2.
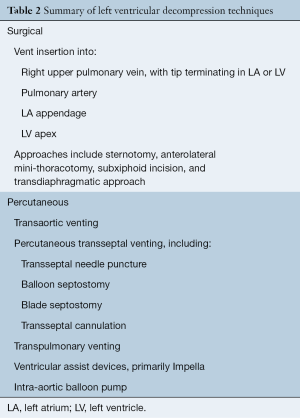
Full table
Direct LV venting: surgical techniques
This technique is most commonly performed via a preexisting sternotomy in patients with post-cardiotomy cardiogenic shock (1,5). Typically, a vent is inserted through the right upper pulmonary vein (PV) with its tip terminating either in the LA or LV, and joined via a Y-connector to the venous access line of the ECMO circuit (5). Other sites for direct vent insertion include the LA appendage, LV apex, and pulmonary artery (11,16). Surgical LV venting provides decompression via large bore tubing that ensures adequate flow rates (17). It also enables direct monitoring of venous and LV arterial blood gases depending on the cannula tip position, which are useful in the detection of other ECMO complications, such as differential hypoxia [in combination with monitoring cerebral near-infrared spectrometry (NIRS)] (17).
Weymann et al. [2014] described LV venting via the right upper pulmonary vein at the time of ECMO cannulation, in 12 adult patients on central VA-ECMO, seven of whom survived to discharge (58%) (17). Three studies of pediatric patients on central VA-ECMO have also shown that surgical LV vents for both elective and emergency indications (as discussed above) result in a rapid improvement in pulmonary edema and left heart distention (11,18,19). In a study by Hacking et al. [2015] of pediatric central VA-ECMO, multivariate linear regression analysis showed that early elective decompression was associated with a shorter duration of ECMO support compared with emergency (late) decompression, although survival was not improved (18).
Several studies have compared elective LV venting to no venting on VA-ECMO support. In a study of 48 adult patients by Schmack et al. [2017], 20 patients on central VA-ECMO support underwent LV decompression via the right upper PV (20). The LV vent was inserted during ECMO cannulation, and repeat echocardiography post venting showed a reduction in LV size compared to patients who did not undergo decompression (20). Patients with a vent also had significantly higher 30-day survival (55% versus 25%, P=0.034), though there was no difference in mortality at 12 months and there were no differences in hepatic, renal, and pulmonary function (20). These results must be interpreted with caution, however, as several important baseline differences between the comparison groups were not controlled for, including younger age in the LV vent group, and a higher proportion of post-cardiotomy shock and IABP insertions in the non LV-vent group (20).
A study of peripheral and central VA-ECMO patients by Tepper et al. [2017] compared surgical LV venting with the Impella LVAD (16). Surgical vents were placed through the LV apex, right superior pulmonary vein, or the pulmonary artery. Unsurprisingly, a higher proportion of central VA-ECMO patients (n=20 versus n=6 in the Impella group) and only two peripheral VA-ECMO patients (versus n=16 in the Impella group) comprised the surgical vent group (16). Patients with LV venting had significantly lower pulmonary arterial diastolic pressure (PADP) at 48 hours than Impella patients, though central venous pressure (CVP) was similar and only five out of 16 surviving LV vent patients had radiological evidence of improved pulmonary edema (16). Survival to 48 hours and 30 days was not significantly different between the groups. However, unadjusted baseline differences in the patient groups (central versus peripheral ECMO, and original indications for ECMO) were some of the many limitations of this study (16). Important complications of LV decompression via sternotomy include bleeding, risk of cardiac trauma and air embolism (16).
Minimally invasive surgical vents
A variety of minimally invasive approaches to LV venting have been described, most commonly via anterolateral mini-thoracotomy. Centofanti et al. [2017] reported on 24 peripheral VA-ECMO patients with concomitant IABP who received a transapical LV vent via a mini-thoracotomy (2). They demonstrated an immediate hemodynamic improvement [reduced CVP, increased achievable ECMO flow, increased mean arterial pressure (MAP) and improved mixed venous oxygen saturation (SvO2)] (2). However, two patients required further surgery for chest wall bleeding from the transapical cannula (2). Keenan and colleagues [2016] described three patients on peripheral VA-ECMO who underwent echocardiographically-guided LV venting for severe pulmonary edema and LV dysfunction, with subsequent hemodynamic improvement and successful weaning (21).
Guirgis et al. [2010] described subxiphoid LV vent placement with a 20 French vent under direct vision in a peripheral VA-ECMO case for acute myocarditis, which was complicated by refractory pulmonary edema and pericardial tamponade (22). The subxiphoid incision used for a pericardial drain was re-opened and extended, and a 20 French vent was inserted into the LV apex under direct vision (22). The patient’s pulmonary edema resolved, a biventricular assist device (BiVAD) was implanted, and she survived to discharge (22).
Other surgical approaches
Eudailey et al. [2015] described a transdiaphragmatic approach to LV venting in a patient who had an intraoperative cardiac arrest during a liver transplant (23). A diaphragmatic incision used for internal cardiac massage and was subsequently used to place a transapical LV vent to treat LV distention and stasis that developed soon after initiating peripheral VA-ECMO (23). Subsequent TOE showed reduced LV distention and increased LV ejection, with reduced vasopressor requirements (23).
In summary, there are a variety of effective surgical approaches to LV venting, although these all have significant risks.
Percutaneous LV venting
Catheter-based approaches
Transaortic vent
Several authors have described a transaortic LV venting technique in peripheral VA-ECMO, where a variably sized (5 to 15 French) catheter is inserted via the femoral artery (contralateral to the ECMO arterial cannula), through the aortic valve and into the apex of the LV (3,24,25). This catheter is then connected to the venous access line of the ECMO circuit. Barbone and colleagues [2011] described this technique on ECMO initiation in three adults using a 7 French pigtail catheter (Johnson & Johnson, New Jersey, USA), with echocardiographic evidence of reduced LV distention (24). Hong et al. [2016] utilized a similar echocardiography-guided technique with a 5 French pigtail catheter (PIG Perfoma, Merit Medical, South Jordan, USA) in seven adult peripheral VA-ECMO patients without procedural complications, and demonstrated significant improvements in LVEDD, LVEF, and MAP (25). Chocron et al. [2013] reported a similar technique utilizing the right subclavian artery and a Carmeda-coated 15 French Bio-Medicus cannula (Medtronic, Minneapolis, USA). Echocardiography (without specification as to whether TTE or TOE was used) demonstrated reduced LV distention and mitral regurgitation (3).
Transaortic venting has the advantage of rapid insertion (with mean procedural times of under 20 minutes) and is less invasive than surgical venting, although the potential risk of damage to the aortic valve over time is unknown (1).
Percutaneous transseptal vent
Percutaneous transseptal venting involves the creation of an atrial septal defect to decompress the LA. Various techniques for this have been described, including transseptal puncture with a Brockenbrough needle; blade septostomy; and balloon septostomy (1). In pediatric patients (e.g., neonates with a patent foramen ovale), balloon atrial septostomy is usually an adequate and straightforward procedure that can be performed at the bedside under echocardiographic guidance (26-28). In older patients, blade septostomy is preferred on the patent and thicker atrial septum (29). Another technique that has been used in both adults and children is transseptal cannulation, in which a catheter is inserted via the femoral vein (under fluoroscopic or echocardiographic guidance) into the LA through the interatrial septum, and then connected to the venous drainage limb of the ECMO circuit to provide decompression (30).
The above strategies in adult and pediatric patients on peripheral VA-ECMO have been shown to reduce PCWP, improve pulmonary edema, reduce LA pressure, improve ECMO output and LVEF, decrease LV distention, and improve ventricular arrhythmias refractory to medical management, as detailed in Tables S1 and S2 (8,10,12,14,26-45).
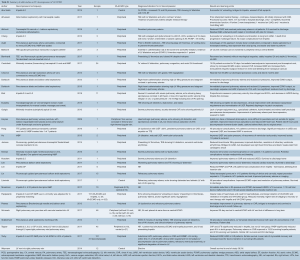
Full table
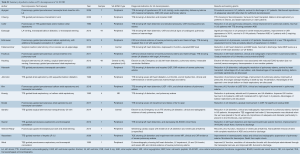
Full table
Atrial septostomy carries a risk of procedural failure. Lin et al. [2017] reported this in two out of 15 patients—the first due to kinking of the cannula during attempted transseptal puncture and venting, and the second due to an inadequately sized ASD for decompression, which required a repeat septostomy (36). Other complications of septostomy include needle perforation of the LA and/or pulmonary vein, pericardial effusion, cardiac tamponade, and ventricular fibrillation (32). Furthermore, atrial septostomy may not prevent LV stasis or thrombosis, and may require subsequent repair (6,46). Alkhouli et al. [2016] found that the majority (50–75%) of iatrogenic ASDs spontaneously close by 6 to 12 months following simple septostomy (14). However, ASDs that persist longer than this have been associated with poorer survival and may require repair (14,40,47).
A potential advantage of atrial stenting over simple blade or balloon septostomy is that it provides a controlled and unrestricted ASD that is less likely to spontaneously close over (1,26). However, in the event of myocardial recovery and attempted weaning from ECMO, open surgical removal is usually required (1). Hence stenting may best be reserved for patients in whom myocardial recovery is not expected, and who will be bridged to either LVAD or transplantation (1). Other complications of atrial stenting include malpositioning or stent dislodgement (due to the thin and mobile atrial septum), LA perforation, damage to nearby structures such as the pulmonary veins, and thromboembolism (1,26,48).
An advantage of transseptal cannulation is that (in common with atrial stenting), a controlled ASD can be tailored to the size of the patient, which has less risk of closure (26). Furthermore, the degree of left heart decompression can be adjusted, by either changing ECMO flow rates or clamping the cannula (26). Finally, due to the elasticity of the atrial septum, the residual ASD following cannula removal is usually small and amenable to device closure under fluoroscopy (26).
Transpulmonary vent
Transpulmonary venting involves the insertion of a variably sized (10 to 15 French) catheter via the right internal jugular vein into the pulmonary artery, which is then connected to the access line of the ECMO circuit (49). Avalli and colleagues [2011] described this technique in an adult patient on peripheral VA-ECMO patient with an LVEF of 10% and LV thrombus (50). Progress TTEs and TOEs by day 30 showed markedly improved LVEF (35–40%) and reduced size of the LV thrombus, which resolved by discharge (50). Fouilloux et al. [2011] described an alternative approach in a 2-year-old girl on VA-ECMO, in whom LV unloading was successfully achieved using a 10 French catheter inserted via the femoral vein and inferior vena cava into the pulmonary trunk (51).
The potential advantages of this technique were first described by von Segesser et al. [2008] in animal models, and include decompression of the pulmonary circulation without reducing right ventricular ejection fraction, fewer complications, and short procedural times, although its relative efficacy remains unknown (50,52).
VADs
Unlike the vents described above, which are all passively driven, VADs actively pump blood from the LV. The most commonly reported VAD used to vent the LV during VA-ECMO support is the Impella (Abiomed, USA).
The Impella is a catheter-based axial flow pump that is introduced into the LV via the femoral artery, with outflow into the aorta (49). It is available in three sizes that provide flows of up to 2.5, 3.5 or 5 L/min (53). Several adult and pediatric studies have shown that the use of an Impella device during VA-ECMO improves LVEDD, clinical and radiographic signs of pulmonary edema, and reduces PCWP and PVR (6,7,9,13,46,54-57). There is limited evidence that this combination improves survival compared with VA-ECMO alone (56).
The Impella device simultaneously decompresses the LV and augments systemic blood flow, which protects the LV from blood stasis and thrombosis (6). For these reasons, the Impella is contraindicated in patients with LV thrombus or significant aortic insufficiency (6). In addition, the Impella flow should be maintained at its lowest effective level in order to minimize the risk of hemolysis (9). The Impella can provide ongoing LV support after weaning from ECMO, thereby extending the time available to decide upon potential bridging strategies (46).
Complications of the Impella device include hemolysis, bleeding, aortic valve insufficiency due to leaflet restriction by the device, limb ischemia, and migration of the pump head into the ascending aorta (e.g., from logrolling for nursing care) (1,49). Pump failure has also been reported in 10% of patients (55). Alkhouli et al. [2016] has also suggested that the degree of LV unloading provided by the Impella may be insufficient for severe LV dysfunction and dilatation (14).
The TandemHeart device is another VAD that decompresses the LV. It comprises a centrifugal pump-assisted bypass between the LA and femoral artery. The access cannula is inserted percutaneously via the femoral vein and transseptally into the LA (49). The return cannula is inserted into the femoral artery, with the tip terminating at the aortic bifurcation (42). Given that this device can provide systemic flows, it can be used as an alternative to VA-ECMO for cardiogenic shock. Li and colleagues [2013] used a TandemHeart combined with an oxygenator to achieve haemodynamic stability in five adult patients in cardiogenic shock (58). Femoral access was used and the presence of the oxygenator avoided the need for transseptal puncture and its associated risks, including cardiac perforation, thromboembolism, and cannula dislodgement (58). In the present review, no study was found that examined the use of the TandemHeart with transseptal LA access as an exclusive strategy for LV decompression on VA-ECMO.
Intra-aortic balloon pump (IABP)
An IABP normally increases aortic diastolic pressures (and coronary perfusion) and reduces LV afterload. Although commonly used in conjunction with peripheral VA-ECMO, it should be noted that diastolic IABP inflation potentially interrupts retrograde peripheral VA-ECMO flow up to 60% of the time (9). The use of an IABP during peripheral VA-ECMO has also been shown to decrease aortic root pressure and coronary blood flow (59). Hence, an IABP may only have potential hemodynamic benefits in centrally cannulated VA-ECMO patients.
Conclusions
Left ventricular volume distention is a significant problem in VA-ECMO patients, with sequelae that include myocardial ischemia, severe pulmonary edema, and intracardiac thrombosis. There is limited data on many key issues, including its incidence, diagnostic criteria and timing for intervention. More research is needed in all these areas, and on the optimal method for LV decompression, given the variety of surgical and percutaneous treatment options that are available.
Acknowledgements
None.
Footnote
Conflicts of Interest: The authors have no conflicts of interest to declare.
References
- Rupprecht L, Florchinger B, Schopka S, et al. Cardiac decompression on extracorporeal life support: a review and discussion of the literature. ASAIO J 2013;59:547-53. [Crossref] [PubMed]
- Centofanti P, Attisani M, La Torre M, et al. Left Ventricular Unloading during Peripheral Extracorporeal Membrane Oxygenator Support: A Bridge To Life In Profound Cardiogenic Shock. J Extra Corpor Technol 2017;49:201-5. [PubMed]
- Chocron S, Perrotti A, Durst C, et al. Left ventricular venting through the right subclavian artery access during peripheral extracorporeal life support. Interact Cardiovasc Thorac Surg 2013;17:187-9. [Crossref] [PubMed]
- Lim HS, Howell N, Ranasinghe A. Extracorporeal Life Support: Physiological Concepts and Clinical Outcomes. J Card Fail 2017;23:181-96. [Crossref] [PubMed]
- Meani P, Gelsomino S, Natour E, et al. Modalities and Effects of Left Ventricle Unloading on Extracorporeal Life support: a Review of the Current Literature. Eur J Heart Fail 2017;19:84-91. [Crossref] [PubMed]
- Cheng A, Swartz MF, Massey HT. Impella to unload the left ventricle during peripheral extracorporeal membrane oxygenation. ASAIO J 2013;59:533-6. [Crossref] [PubMed]
- Truby LK, Takeda K, Mauro C, et al. Incidence and Implications of Left Ventricular Distention During Venoarterial Extracorporeal Membrane Oxygenation Support. ASAIO J 2017;63:257-65. [Crossref] [PubMed]
- Litwiński P, Demkow M, Sobieszczanska M, et al. Transseptal balloon atrial septostomy for decompression of the left atrium during extracorporeal membrane oxygenation support as a "bridge to transplantation" in dilated cardiomyopathy. Postepy Kardiol Interwencyjnej 2017;13:72-4. [Crossref] [PubMed]
- Eliet J, Gaudard P, Zeroual N, et al. Effect of Impella During Veno-Arterial Extracorporeal Membrane Oxygenation on Pulmonary Artery Flow as Assessed by End-Tidal Carbon Dioxide. ASAIO J 2018;64:502-7. [Crossref] [PubMed]
- Eckhauser AW, Jones C, Witte MK, et al. Transthoracic echocardiographic predictors of left atrial hypertension in patients on venoarterial extracorporeal membrane oxygenation. World J Pediatr Congenit Heart Surg 2014;5:67-9. [Crossref] [PubMed]
- Falkensammer CB, Heinle JS, Chang AC. Serial plasma BNP levels in assessing inadequate left ventricular decompression on ECMO. Pediatr Cardiol 2008;29:808-11. [Crossref] [PubMed]
- Sidebotham D, Allen S, McGeorge A, et al. Catastrophic left heart distension following initiation of venoarterial extracorporeal membrane oxygenation in a patient with mild aortic regurgitation. Anaesth Intensive Care 2012;40:568-9. [PubMed]
- Lim HS. The Effect of Impella CP on Cardiopulmonary Physiology During Venoarterial Extracorporeal Membrane Oxygenation Support. Artif Organs 2017;41:1109-12. [Crossref] [PubMed]
- Alkhouli M, Narins CR, Lehoux J, et al. Percutaneous Decompression of the Left Ventricle in Cardiogenic Shock Patients on Venoarterial Extracorporeal Membrane Oxygenation. J Card Surg 2016;31:177-82. [Crossref] [PubMed]
- Ong CS, Hibino N. Left heart decompression in patients supported with extracorporeal membrane oxygenation for cardiac disease. Postepy Kardiol Interwencyjnej 2017;13:1-2. [Crossref] [PubMed]
- Tepper S, Masood MF, Baltazar Garcia M, et al. Left Ventricular Unloading by Impella Device Versus Surgical Vent During Extracorporeal Life Support. Ann Thorac Surg 2017;104:861-7. [Crossref] [PubMed]
- Weymann A, Schmack B, Sabashnikov A, et al. Central extracorporeal life support with left ventricular decompression for the treatment of refractory cardiogenic shock and lung failure. J Cardiothorac Surg 2014;9:60. [Crossref] [PubMed]
- Hacking DF, Best D, d'Udekem Y, et al. Elective decompression of the left ventricle in pediatric patients may reduce the duration of venoarterial extracorporeal membrane oxygenation. Artif Organs 2015;39:319-26. [Crossref] [PubMed]
- Sandrio S, Springer W, Karck M, et al. Extracorporeal life support with an integrated left ventricular vent in children with a low cardiac output. Cardiol Young 2014;24:654-60. [Crossref] [PubMed]
- Schmack B, Seppelt P, Weymann A, et al. Extracorporeal life support with left ventricular decompression-improved survival in severe cardiogenic shock: results from a retrospective study. PeerJ 2017;5:e3813. [Crossref] [PubMed]
- Keenan JE, Schechter MA, Bonadonna DK, et al. Early Experience with a Novel Cannulation Strategy for Left Ventricular Decompression during Nonpostcardiotomy Venoarterial ECMO. ASAIO J 2016;62:e30-4. [Crossref] [PubMed]
- Guirgis M, Kumar K, Menkis AH, et al. Minimally invasive left-heart decompression during venoarterial extracorporeal membrane oxygenation: an alternative to a percutaneous approach. Interact Cardiovasc Thorac Surg 2010;10:672-4. [Crossref] [PubMed]
- Eudailey KW, Yi SY, Mongero LB, et al. Trans-diaphragmatic left ventricular venting during peripheral venous-arterial extracorporeal membrane oxygenation. Perfusion 2015;30:701-3. [Crossref] [PubMed]
- Barbone A, Malvindi PG, Ferrara P, et al. Left ventricle unloading by percutaneous pigtail during extracorporeal membrane oxygenation. Interact Cardiovasc Thorac Surg 2011;13:293-5. [Crossref] [PubMed]
- Hong TH, Byun JH, Lee HM, et al. Initial Experience of Transaortic Catheter Venting in Patients with Venoarterial Extracorporeal Membrane Oxygenation for Cardiogenic Shock. ASAIO J 2016;62:117-22. [PubMed]
- Hlavacek AM, Atz AM, Bradley SM, et al. Left atrial decompression by percutaneous cannula placement while on extracorporeal membrane oxygenation. J Thorac Cardiovasc Surg 2005;130:595-6. [Crossref] [PubMed]
- Johnston TA, Jaggers J, McGovern JJ, et al. Bedside transseptal balloon dilation atrial septostomy for decompression of the left heart during extracorporeal membrane oxygenation. Catheter Cardiovasc Interv 1999;46:197-9. [Crossref] [PubMed]
- Koenig PR, Ralston MA, Kimball TR, et al. Balloon atrial septostomy for left ventricular decompression in patients receiving extracorporeal membrane oxygenation for myocardial failure. J Pediatr 1993;122:S95-9. [Crossref] [PubMed]
- Cheung MM, Goldman AP, Shekerdemian LS, et al. Percutaneous left ventricular "vent" insertion for left heart decompression during extracorporeal membrane oxygenation. Pediatr Crit Care Med 2003;4:447-9. [Crossref] [PubMed]
- Bernhardt AM, Hillebrand M, Yildirim Y, et al. Percutaneous left atrial unloading to prevent pulmonary oedema and to facilitate ventricular recovery under extracorporeal membrane oxygenation therapy. Interact Cardiovasc Thorac Surg 2018;26:4-7. [Crossref] [PubMed]
- Alhussein M, Osten M, Horlick E, et al. Percutaneous left atrial decompression in adults with refractory cardiogenic shock supported with veno-arterial extracorporeal membrane oxygenation. J Card Surg 2017;32:396-401. [Crossref] [PubMed]
- Dahdouh Z, Roule V, Lognone T, et al. Atrial septostomy in cardiogenic shock related to H1N1 infection. Acute Card Care 2013;15:7-9. [Crossref] [PubMed]
- Dahdouh Z, Roule V, Sabatier R, et al. Extra-corporeal life support, transradial thrombus aspiration and stenting, percutaneous blade and balloon atrioseptostomy, all as a bridge to heart transplantation to save one life. Cardiovasc Revasc Med 2012;13:241-5. [Crossref] [PubMed]
- Jumean M, Pham DT, Kapur NK. Percutaneous bi-atrial extracorporeal membrane oxygenation for acute circulatory support in advanced heart failure. Catheter Cardiovasc Interv 2015;85:1097-9. [Crossref] [PubMed]
- Lee SI, Lee SY, Choi CH, et al. Left Heart Decompression in Acute Complicated Myocardial Infarction During Extracorporeal Membrane Oxygenation. J Intensive Care Med 2017;32:405-8. [Crossref] [PubMed]
- Lin YN, Chen YH, Wang HJ, et al. Atrial Septostomy for Left Atrial Decompression During Extracorporeal Membrane Oxygenation by Inoue Balloon Catheter. Circ J 2017;81:1419-23. [Crossref] [PubMed]
- Peterss S, Pfeffer C, Reichelt A, et al. Extracorporeal life support and left ventricular unloading in a non-intubated patient as bridge to heart transplantation. Int J Artif Organs 2013;36:913-6. [Crossref] [PubMed]
- Aiyagari RM, Rocchini AP, Remenapp RT, et al. Decompression of the left atrium during extracorporeal membrane oxygenation using a transseptal cannula incorporated into the circuit. Crit Care Med 2006;34:2603-6. [Crossref] [PubMed]
- Cofer BR, Warner BW, Stallion A, et al. Extracorporeal membrane oxygenation in the management of cardiac failure secondary to myocarditis. J Pediatr Surg 1993;28:669-72. [Crossref] [PubMed]
- Eastaugh LJ, Thiagarajan RR, Darst JR, et al. Percutaneous left atrial decompression in patients supported with extracorporeal membrane oxygenation for cardiac disease. Pediatr Crit Care Med 2015;16:59-65. [Crossref] [PubMed]
- Kim HE, Jung JW, Shin YR, et al. Left Atrial Decompression by Percutaneous Left Atrial Venting Cannula Insertion during Venoarterial Extracorporeal Membrane Oxygenation Support. Korean J Thorac Cardiovasc Surg 2016;49:203-6. [Crossref] [PubMed]
- Kredel M, Kunzmann S, Schlegel PG, et al. Double Peripheral Venous and Arterial Cannulation for Extracorporeal Membrane Oxygenation in Combined Septic and Cardiogenic Shock. Am J Case Rep 2017;18:723-7. [Crossref] [PubMed]
- Swartz MF, Smith F, Byrum CJ, et al. Transseptal catheter decompression of the left ventricle during extracorporeal membrane oxygenation. Pediatr Cardiol 2012;33:185-7. [Crossref] [PubMed]
- Veeram Reddy SR, Guleserian KJ, Nugent AW. Transcatheter removal of atrial septal stent placed to decompress left atrium with VA ECMO. Catheter Cardiovasc Interv 2015;85:1021-5. [Crossref] [PubMed]
- Ward KE, Tuggle DW, Gessouroun MR, et al. Transseptal decompression of the left heart during ECMO for severe myocarditis. Ann Thorac Surg 1995;59:749-51. [Crossref] [PubMed]
- Abu Saleh WK, Mason P, Al Jabbari O, et al. Successful use of surgically placed impella 5.0: And central extracorporeal membrane oxygenation circuit in a patient with postcardiotomy shock. Tex Heart Inst J 2015;42:569-71. [Crossref] [PubMed]
- Schueler R, Öztürk C, Wedekind JA, et al. Persistence of Iatrogenic Atrial Septal Defect After Interventional Mitral Valve Repair With the MitraClip System: A Note of Caution. JACC Cardiovasc Interv 2015;8:450-9. [Crossref] [PubMed]
- Haynes S, Kerber RE, Johnson FL, et al. Left heart decompression by atrial stenting during extracorporeal membrane oxygenation. Int J Artif Organs 2009;32:240-2. [Crossref] [PubMed]
- Meani P, Natour E, Pappalardo F, et al. Left ventricle unloading in veno-arterial ECMO support: Literature review for an unanswered dilemma. Eur J Heart Fail 2017;19:64-6.
- Avalli L, Maggioni E, Sangalli F, et al. Percutaneous left-heart decompression during extracorporeal membrane oxygenation: an alternative to surgical and transeptal venting in adult patients. ASAIO J 2011;57:38-40. [Crossref] [PubMed]
- Fouilloux V, Lebrun L, Mace L, et al. Extracorporeal membranous oxygenation and left atrial decompression: a fast and minimally invasive approach. Ann Thorac Surg 2011;91:1996-7. [Crossref] [PubMed]
- von Segesser L, Dembitsky W, Ferrari E, et al. A simple way to decompress the left ventricle during veno-arterial bypass. Thorac Cardiovasc Surg 2008;56:337-41. [PubMed]
- Cena M, Karam F, Ramineni R, et al. New Impella Cardiac Power Device Used in Patient with Cardiogenic Shock due to Nonischemic Cardiomyopathy. Int J Angiol 2016;25:258-62. [PubMed]
- Koeckert MS, Jorde UP, Naka Y, et al. Impella LP 2.5 for left ventricular unloading during venoarterial extracorporeal membrane oxygenation support. J Card Surg 2011;26:666-8. [Crossref] [PubMed]
- Moazzami K, Dolmatova EV, Cocke TP, et al. Left Ventricular Mechanical Support with the Impella during Extracorporeal Membrane Oxygenation. J Tehran Heart Cent 2017;12:11-4. [PubMed]
- Pappalardo F, Schulte C, Pieri M, et al. Concomitant implantation of Impella® on top of veno-arterial extracorporeal membrane oxygenation may improve survival of patients with cardiogenic shock. Eur J Heart Fail 2017;19:404-12. [Crossref] [PubMed]
- Vlasselaers D, Desmet M, Desmet L, et al. Ventricular unloading with a miniature axial flow pump in combination with extracorporeal membrane oxygenation. Intensive Care Med 2006;32:329-33. [Crossref] [PubMed]
- Li YW, Rosenblum WD, Gass AL, et al. Combination use of a TandemHeart with an extracorporeal oxygenator in the treatment of five patients with refractory cardiogenic shock after acute myocardial infarction. Am J Ther 2013;20:213-8. [Crossref] [PubMed]
- Bělohlávek J, Mlček M, Huptych M, et al. Coronary versus carotid blood flow and coronary perfusion pressure in a pig model of prolonged cardiac arrest treated by different modes of venoarterial ECMO and intraaortic balloon counterpulsation. Crit Care 2012;16:R50. [Crossref] [PubMed]