A reassessment of tracheal substitutes—a systematic review
Introduction
The “illusory simplicity” of developing a tracheal substitute has been confirmed time and time again over the past 75 years (1). Since the turn of the century, the field has seen the development of innovative techniques and suffered setbacks through premature clinical translation of understudied procedures. Four primary methodologies have emerged: tracheal allotransplantation, autologous tissue reconstruction, bioprosthetic reconstruction, and tissue engineered reconstruction. Entirely prosthetic circumferential tracheal substitutes have universally failed in clinical practice since the 1960s and will not be discussed (2). Each of the four methodologies listed above contain unique advantages and disadvantages (Table 1). Herein, we will describe the unique challenges of airway reconstruction and perform a systematic assessment of current clinical approaches.
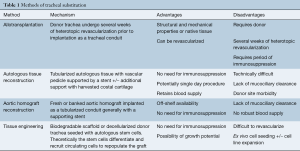
Full table
Clinical need
Multiple etiologies including trauma, congenital malformation, neoplastic disease, and prolonged intubation can results in significant injury to the trachea that requires resection and reconstruction. Unlike pathology of other organs such as the liver, lungs, and blood vessels the trachea can generally be repaired primarily and rarely requires whole organ replacement. Through advancements over the past century approximately one-half or 5 to 6 cm of the trachea can be safely removed and primarily repaired in the adult. In a child, the safe length of resection with primary reconstruction is reduced to one-third of the trachea (3,4). Only in rare cases of uncommon malignancies or particularly large aerodigestive fistulas is an alternative to primary repair required. Even in these cases the resulting defect after resection is not always circumferential and may be amenable to a patch repair (5). For patients with benign disease of the trachea, stents and T tubes can be used for palliation. However, these foreign bodies are not without risk of erosion, infection, and fistula formation resulting in morbidity and catastrophic bleeding when blood vessels are involved. For the rare patient with a circumferential airway defect whose length and complexity preclude primary repair, a safe and effective tracheal substitute would be of benefit.
Challenges of the airway
The trachea serves two primary functions (I) an airtight and mechanically stable passage from the larynx to the bronchus; and (II) facilitator for clearance of secretions. The first function is accomplished through a tubular design that is supported by anterior cartilaginous rings and longitudinal muscle that together provide lateral rigidity and longitudinal flexibility. The second function is accomplished through specialized epithelium with synchronized cilia to move secretions up the mucociliary escalator (6,7).
The greatest challenges in tracheal replacement are restoration of blood supply and the interface with the outside world, e.g., exposure to bacteria and fungus. The trachea depends on segmental blood supply via small vessels that penetrate in between cartilage rings to provide blood to the luminal mucosal lining. Any transplanted trachea, autologous substitute, or tissue engineered scaffold would have to rapidly develop its own blood supply to support cellular metabolism. Likewise, the constant exposure of the trachea to the outside world represents a major hurdle due to desiccation, exposure to toxins, and microorganisms. Unlike the sterile environment of a blood vessel or hip joint, any foreign body implanted in the airway will eventually be exposed to pathogens. When superinfection occurs, the anastomosis will be compromised and if not addressed can lead to catastrophic dehiscence (1,2).
Methods
Literature search strategy
Electronic searches were performed using PubMed/Medline for articles published form January 2000 to September 2017. We used “tracheal replacement” or “tracheal substitute” or “tracheal regeneration” or “tracheal tissue engineering” or “tracheal transplantation” as key words or medical subject heading (MeSH) terms. All identified articles were systematically assessed using the inclusion and exclusion criteria listed below. The reference lists of articles matching inclusion criteria were then reviewed for further identification of potentially relevant studies.
Selection criteria
Studies in which patients underwent circumferential or near-circumferential (>270°) replacement of the trachea were included in the study. Animal model and non-clinical studies were excluded. All publications were limited to the English language. Abstracts, conference presentations, editorials, and expert opinions were excluded. Reviews in which new patient information was reported were included. In addition, all retracted articles were excluded from analysis.
Data extraction
Article text, figures, and tables were reviewed. Two investigators (B Udelsman and HC Ott) reviewed studies selected through exclusion and inclusions criteria. Discrepancies between the two reviewers were resolved by discussion and consensus achieved.
Results
Quantity and quality of clinical studies
Using specified search criteria, a total of 2,168 articles were identified. After applying the inclusion and exclusion criteria the number of articles was reduced to 22. Manual review of references did not reveal any additional articles. One article was retracted after publication and thus not included in analysis. We have included three articles published by groups led by Dr. Macchiarini; however, given recent concerns regarding scientific misconduct we view these results with caution and skepticism (8,9). Further discussion is included in the section on tissue engineering below. The 21 included articles consisted of a mix of case series, case reports, and a review which included clinical updates. In sum, a total of 41 patients have undergone a reported repair with a tracheal substitute and the result of that repair is reported herein (Figure 1).
Tracheal allotransplantation
As discussed above the trachea receives its segmental blood supply through numerous perforating vessels fed by many small tracheal esophageal vessels which preclude standard vascular anastomosis and single-stage transplantation. If a donor trachea is implanted without first reestablishing blood supply the graft will inevitably fail. To develop a sufficient blood supply to a transplanted trachea, Delaere et al. have proposed a two-stage procedure (10). The donor trachea is first implanted in the forearm of the recipient after being wrapped in a fasciocutaneous flap perfused by the radial vascular pedicle. To prevent rejection the recipient is placed on immunosuppressants while the donor trachea is revascularized by ingrowth of recipient vessels based of the radial vascular pedicle. After sufficient ingrowth, the tracheal allograft along with perfusing vascular pedicle can be explanted from the heterotopic position and implanted in the orthotopic position. The blood supply is reestablished by anastomosis of the pedicle to local arteries and veins such as the superior thyroid artery and internal jugular vein. Eventually the mucosa of the donor trachea can be repopulated by recipient cells creating a chimeric graft and allowing for withdrawal of immunosuppressants (2).
In 2008, Delaere et al. reported on the successful implantation of a tracheal allograft using the above method (10). Since that time the same group has reported on a total of six patients treated with tracheal allografts (2). The primary complications have been graft rejection after withdrawal of immunosuppression leading to partial loss of the allograft in three patients. Overtime the reported technique has evolved to promote donor repopulation of the mucosa and prevent stricture after withdrawal of immunosuppressants (2,11,12). The most recent reported protocol describes a 4-month long period of heterotopic implantation during which inosculation takes place. To promote this process, small incisions can be made in the intercartilaginous ligaments to facilitate ingrowth of recipient donor vessels. After sufficient revascularization, graft-mucosa chimerism is generated through the denuding of donor mucosal lining at the graft midpoint and placement of full thickness recipient buccal mucosal grafts. The composite graft can then be implanted with gradual tapering of immunosuppressants starting at 15 months post-operatively. The donor chondrocytes remain intact as they lack a direct blood supply and are protected from host immunodetection through encasement within a dense extracellular matrix (10).
While tracheal transplantation represents an effective method of tracheal replacement, allotransplantation does have its limitations. First, it requires several months of heterotopic development of the graft which precludes an emergent or urgent transplantation. Second, the method requires an extended period of immunosuppression which introduces the risk of opportunistic infection and limits its use in cases of malignancy. Of the six patients in which allotransplantation have been attempted, all but one had benign pathology. The single patient with malignancy possessed a low-grade laryngotracheal chondrosarcoma which had demonstrated slow growth over a period of 10 years (2). In patients with high-grade malignancies the need for immunosuppression during the heterotopic revascularization phase may be prohibitive due to oncologic implications. Finally, due to higher metabolic demands and difficulty achieving rapid revascularization the membranous wall of the donor trachea undergoes avascular necrosis after heterotopic transplantation. For this reason, the membranous wall of the native trachea is recreated using recipient skin flaps. The squamous epithelium of the neo-membranous trachea can lead to impaired mucociliary clearance of secretions, and colonization with abnormal flora in this section of the trachea.
Autologous tissue reconstruction
Multiple autologous tissue sources have been used for tracheal reconstruction including pulmonary, skin, and forearm flaps (Table 2). Despite the different tissues used, these methods of repair and reconstruction share similar underlying methodology (13-16). No matter which tissue is used, the goal is to provide a well perfused pedicled graft which can be tubularized into a neo-trachea. In many cases, these grafts are supported by a temporary or permanent stenting. They can be additionally supported through implantation of cartilaginous rings harvested and fashioned from costal cartilage. Fabre et al. reported the largest series using this technique which included 12 patients (15). In these patients, repair was performed in a single staged, albeit lengthy (12 to 17 hours) procedure, through use of a radial fasciocutaneous flap supported both by strips of harvested costal cartilage and by a temporary luminal stent.
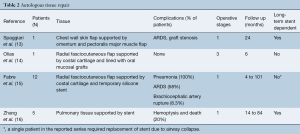
Full table
While innovative in their approach, the results of autologous repair have been mixed. There is generally a need for at least short-term stenting due malacia and stenosis, which negates some of the benefit of airway reconstruction. Second, through this technique it is not possible to regenerated airway epithelium and thus the mucociliary escalator is lost. Patients with this repair can become dependent on frequent bronchoscopic clearance and require a robust cough to help clear the airway. In the 12 patient series reported by Fabre et al., seven developed acute respiratory distress syndrome and two patients were tracheostomy dependent. For this reason, the authors have recommended not to perform this approach of airway reconstruction on patients with diaphragmatic dysfunction or those that require extension of the airway repair into the bronchus (15). A possible exception to the lack of airway epithelium is described by Olias et al. who harvest oral mucosal grafts to line their lumen of their neo-trachea. Unfortunately, this requires a staged procedure and is associated with some degree of donor site morbidity (14).
Bioprosthetic reconstruction
Aortic homografts have been used in valve and arch repair since the 1960s (17). Use in the repair of tracheal defects began in late 1990s and has continued throughout the past two decades. Likewise, acellular dermal matrix has been adopted in for use in airway repair over the 10 years (5). The majority of bioprosthetic repairs have been patch repairs in which the bioprosthetic material is not used for circumferential repair or creation of a neo-trachea. Nonetheless, since 2001, the use of aortic interpositional grafts in circumferential repairs has been reported by four different groups of investigators and used in 11 individual patients (18-23). Extracellular dermal matrix has been used only once in circumferential repair and was associated with graft migration requiring revision (24). The advantage of this type of repair is in the commercial and potentially off-shelf availability. The downsides are the lack of a respiratory epithelium to aid in mucociliary clearance of secretions, and the relative pliability of grafts typically requiring stenting to prevent malacia. In addition, it is unclear how the graft becomes repopulated with host cells and how blood supply is reestablished.
The first reported use an aortic homograft in repair of a circumferential defect was by Hoffman et al. in which a tubularized interpositional aortic homograft was used as a temporary conduit in a patient with a tracheal dehiscence after lung transplant. No stent was used in the aortic homograft reconstruction and patency of the airway was maintained only by continuous positive pressure ventilation. The patient underwent successful retransplant 3 days later at which time the homograft was resected (18). Permanent use of a tubularized aortic graft in the construction of a neo-trachea was first reported by Azorin et al. in 2006 (19). After extensive animal studies the group elected to use an aortic autograft to reconstruct the trachea in a patient after resection of a 7-cm squamous cell tumor. While technically the repair was with autologous tissue rather than a homograft, the repair was dissimilar to the reports of autologous tissue repair detailed in the above section in that no vascular pedicle was transplanted with the graft. In their report, a patient underwent successful repair with the aortic conduit supported by a stent and could be extubated within 12 hours. The patient required further stenting over the post-operative period, but was eventually able to maintain a patent airway without stents by 3 months after his initial surgery. Unfortunately, at 6 months after his initial surgery the patient developed severe bilateral pneumonia and expired. Bronchoscopy near the time of death demonstrated no evidence of dehiscence or perforation of the graft (19).
The use of an aortic homograft as a permanent neo-trachea was attempted in 2009 by Davidson et al. (21). In their report, a tracheal dehiscence after take down of a tracheoesophageal fistula was repaired using banked aortic homograft and Montgomery tube for stenting. The patient could be extubated postoperatively; however, went into cardiac arrest on post-operative day 10 and on autopsy was found to have a dehiscence of the proximal anastomosis. The authors hypothesize that the use of the Montgomery tube instead an endotracheal stent provided inadequate support to the repair and contributed to the dehiscence and secondary mediastinitis.
The largest study utilizing aortic homograft was reported by Wurtz et al., in which homografts that were supported internally by a stent and externally by a muscle flap buttress were used to reconstruct the airway in six patients with large mucoepidermoid and adenoid cystic carcinomas (20,22). Five of the six patients who underwent repair could be extubated; however, all remained stent dependent and 50% encountered major complications such as anastomotic dehiscence, sternal dehiscence, and fungal infection of the graft.
The most recent reports of aortic homograft repair have come from Martinod et al. Repair was accomplished in two patients with benign laryngeal tracheal stenosis through aortic homograft reconstruction supported by Nitinol stents and buttressed with strap muscle (23). Importantly, Martinod preserved the membranous portion of the native trachea in their repair and used aortic grafts preserved at −80 °C rather than the standard −150 °C. The group reported eventual stent removal between 15 and 39 months with the regeneration of cartilage within the graft. The authors proposed an “in vivo tissue engineering” mechanism in which retained growth and angiogenic factors with within the donor extracellular matrix are released and lead to the migration, proliferation, and differentiation of host cells (23). While these early results are exciting the robustness of this type of repair and the underlying mechanism require further study and validation, especially if it is to be used in patients with underlying malignancy, in which the repair will be further stressed by adjuvant therapy.
Tissue engineering
Since its popularization by Langer and Vacanti in the 1990s, tissue engineering has held the offer of a potential limitless supply of autologous tissue for organ transplantation (25). The method employs aspects of bioengineering to promote tissue regeneration. While multiple frameworks for this process have been proposed, in general the method involves the implantation of a biodegradable scaffold seeded with previously harvested host cells. In a bioreactor or after implantation the host stem cells differentiate into mature cells that repopulate the biodegradable scaffold and eventually replace it through deposition of a new extracellular matrix. Alternately, in some situations the seeded cells may orchestrate the recruitment and repopulation of the scaffold by circulating host cells through release of various cytokines and chemoattractants (26). Tissue engineering is a particularly attractive method in a pediatric population, as a completely autologous organ may demonstrate growth potential sparing patients initial size miss-matches or reoperation procedures as they grow.
Despite the high hopes for tracheal tissue engineering, the field has been setback by controversy and scientific misconduct. In 2008, Macchiarini et al. published on the first clinical transplantation of a tissue-engineered trachea (27). This work was followed up with several more reports by the same group notably in 2011 and 2014 (28,29). Unfortunately, it has become clear after both internal and external investigations of Dr. Macchiarini’s work that the reported success of this technique was overstated and many of the patients who underwent these procedures developed devastating complications (2,8,30,31).
Aside from the work performed by the Macchiarini group, two pediatric patients have undergone treatment with tissue engineered tracheal conduits (32-34). Both patients suffered from severe congenital airway defects and had undergone multiple prior corrective procedures. The first patient underwent repair of a recurrent stent related tracheal aortic fistula in 2010. At the time of surgery, a bone-marrow aspirate was performed and mononuclear cells isolated. A suspension of these cells was used to seed a cadaveric tracheal homograft. In addition, the tracheal rings of the graft were injected with tissue transforming growth factor beta and the entire construct soaked in human recombinant erythropoietin and granulocyte colony stimulating factor prior to implantation (32). Despite the need for multiple stenting and bronchoscopic procedures, especially within the first year of implantation, at long-term follow-up the graft remained patent with evidence of a ciliated epithelial cell layer at 4 years (33).
An additional pediatric patient with multiple congenital abnormalities of the airway as well as multiple prior interventions underwent treatment with a tissue engineered tracheal conduit in 2012 (23). In this case, the decellularized cadaveric tracheal graft was seeded over a period of 48 hours in a bioreactor with expanded bone marrow-derived mesenchymal stromal cells as well as autologous respiratory epithelial cell obtained from mucosal biopsies of the nasal septum. Due to a lack of sufficient omentum, surgeons were unable to support with graft with a tissue buttress and chose not to support the graft with a stent given concerns of inducing an overly robust inflammatory process. While the initial postoperative course after transplantation was uncomplicated with evidence of only mild malacia, the patient deteriorated on post-operative day 15 and suffered a prolonged respiratory arrest thought to be secondary to airway collapse and external compression. While the patient was successfully resuscitated, severe hypoxic brain injury had occurred and the decision was made to withdraw care.
While tissue engineering approaches to airway reconstruction may have had some success, both the reported and unreported failures support further laboratory and animal model based research. Based on clinical data available to date, it remains unclear how the implanted scaffold becomes revascularized or how seeded cells orchestrate the repopulation of the decellularized tracheal graft.
Discussion
Finding an ideal airway substitute remains an elusive target. A viable airway graft that serves as a competent conduit for respiration, and provides mucociliary clearance of secretions has yet to be developed. While each of the four modalities of airway replacement described herein have had some clinical success, none of these techniques are ideal or currently ready for widespread clinical translation. Based on the literature reviewed, we firmly conclude that to produce a clinically valid airway substitute, further laboratory and large animal models are needed to address several unsolved issues. Minimal design criteria for the success of such a graft are: (I) immediate perfusability upon implantation via direct vascular anastomosis, or rapid revascularization of a non-pedicled conduit; (II) sufficient mechanical stability to enable postoperative extubation and long-term freedom from intraluminal stenting; (III) lack of erosion into surrounding structures; (IV) establishment of a sufficient barrier function to prevent infection of graft material and surrounding structures; (V) formation of an epithelial lining that is compatible with formation and possibly transport of a thin layer of mucous to prevent bacterial overgrowth, and retention of secretions; and (VI) in case of pediatric application a graft that can adapt to changes of physiologic need. Finally, as the technologies focused on tracheal substitution evolve rigorous and transparent evaluation of laboratory and experimental data needs to occur to ensure safety and efficacy prior to clinical translation.
Acknowledgements
None.
Footnote
Conflicts of Interest: The authors have no conflicts of interest to declare.
References
- Grillo HC. Tracheal replacement: a critical review. Ann Thorac Surg 2002;73:1995-2004. [Crossref] [PubMed]
- Delaere P, Van Raemdonck D. Tracheal replacement. J Thorac Dis 2016;8:S186-96. [PubMed]
- Grillo HC. Surgery of the trachea. Curr Probl Surg 1970.3-59. [PubMed]
- Grillo HC. Notes on the windpipe. Ann Thorac Surg 1989;47:9-26. [Crossref] [PubMed]
- Udelsman BV, Eaton J, Muniappan A, et al. Repair of large airway defects with bioprosthetic materials. J Thorac Cardiovasc Surg 2016;152:1388-97. [Crossref] [PubMed]
- Belsey R. Resection and reconstruction of the intrathoracic trachea. Br J Surg 1950;38:200-5. [Crossref] [PubMed]
- Hamilton N, Bullock AJ, Macneil S, et al. Tissue engineering airway mucosa: a systematic review. Laryngoscope 2014;124:961-8. [Crossref] [PubMed]
- Cyranoski D. Investigations launched into artificial tracheas. Nature 2014;516:16-7. [Crossref] [PubMed]
- Delaere PR, Van Raemdonck D. The trachea: the first tissue-engineered organ? J Thorac Cardiovasc Surg 2014;147:1128-32. [Crossref] [PubMed]
- Delaere P, Vranckx J, Verleden G, et al. Tracheal allotransplantation after withdrawal of immunosuppressive therapy. N Engl J Med 2010;362:138-45. [Crossref] [PubMed]
- Delaere PR, Vranckx JJ, Meulemans J, et al. Learning curve in tracheal allotransplantation. Am J Transplant 2012;12:2538-45. [Crossref] [PubMed]
- Delaere PR, Vranckx JJ, Den Hondt M, et al. Tracheal allograft after withdrawal of immunosuppressive therapy. N Engl J Med 2014;370:1568-70. [Crossref] [PubMed]
- Spaggiari L, Calabrese LS, D'Aiuto M, et al. Successful subtotal tracheal replacement (using a skin/omental graft) for dehiscence after a resection for thyroid cancer. J Thorac Cardiovasc Surg 2005;129:1455-6. [Crossref] [PubMed]
- Olias J, Millan G, da Costa D. Circumferential tracheal reconstruction for the functional treatment of airway compromise. Laryngoscope 2005;115:159-61. [Crossref] [PubMed]
- Fabre D, Kolb F, Fadel E, et al. Successful tracheal replacement in humans using autologous tissues: an 8-year experience. Ann Thorac Surg 2013;96:1146-55. [Crossref] [PubMed]
- Zhang S, Liu Z. Airway Reconstruction with Autologous Pulmonary Tissue Flap and an Elastic Metallic Stent. World J Surg 2015;39:1981-5. [Crossref] [PubMed]
- Stelzer P, Elkins RC. Homograft valves and conduits: applications in cardiac surgery. Curr Probl Surg 1989;26:381-452. [Crossref] [PubMed]
- Hoffman TM, Gaynor JW, Bridges ND, et al. Aortic homograft interposition for management of complete tracheal anastomotic disruption after heart-lung transplantation. J Thorac Cardiovasc Surg 2001;121:587-8. [Crossref] [PubMed]
- Azorin JF, Bertin F, Martinod E, et al. Tracheal replacement with an aortic autograft. Eur J Cardiothorac Surg 2006;29:261-3. [Crossref] [PubMed]
- Wurtz A, Porte H, Conti M, et al. Tracheal replacement with aortic allografts. N Engl J Med 2006;355:1938-40. [Crossref] [PubMed]
- Davidson MB, Mustafa K, Girdwood RW. Tracheal replacement with an aortic homograft. Ann Thorac Surg 2009;88:1006-8. [Crossref] [PubMed]
- Wurtz A, Porte H, Conti M, et al. Surgical technique and results of tracheal and carinal replacement with aortic allografts for salivary gland-type carcinoma. J Thorac Cardiovasc Surg 2010;140:387-93.e2. [Crossref] [PubMed]
- Martinod E, Paquet J, Dutau H, et al. In Vivo Tissue Engineering of Human Airways. Ann Thorac Surg 2017;103:1631-40. [Crossref] [PubMed]
- Bolton WD, Ben-Or S, Hale AL, et al. Reconstruction of a Long-Segment Tracheal Defect Using an AlloDerm Conduit. Innovations (Phila) 2017;12:137-9. [Crossref] [PubMed]
- Langer R, Vacanti JP. Tissue engineering. Science 1993;260:920-6. [Crossref] [PubMed]
- Roh JD, Sawh-Martinez R, Brennan MP, et al. Tissue-engineered vascular grafts transform into mature blood vessels via an inflammation-mediated process of vascular remodeling. Proc Natl Acad Sci U S A 2010;107:4669-74. [Crossref] [PubMed]
- Macchiarini P, Jungebluth P, Go T, et al. Clinical transplantation of a tissue-engineered airway. Lancet 2008;372:2023-30. [Crossref] [PubMed]
- Jungebluth P, Alici E, Baiguera S, et al. Tracheobronchial transplantation with a stem-cell-seeded bioartificial nanocomposite: a proof-of-concept study. Lancet 2011;378:1997-2004. [Crossref] [PubMed]
- Gonfiotti A, Jaus MO, Barale D, et al. The first tissue-engineered airway transplantation: 5-year follow-up results. Lancet 2014;383:238-44. [Crossref] [PubMed]
- Vogel G. Trachea transplants test the limits. Science 2013;340:266-8. [Crossref] [PubMed]
- Heckscher S, Carlberg I, Gahmberg C. Karolinska Institutet and the Macchiarini case. In: Heckscher S, Carlberg I, Gahmberg C. editors. English and Swedish. An external inquiry. Stockholm: Karolinska institutet, 2016:1-20.
- Elliott MJ, De Coppi P, Speggiorin S, et al. Stem-cell-based, tissue engineered tracheal replacement in a child: a 2-year follow-up study. Lancet 2012;380:994-1000. [Crossref] [PubMed]
- Hamilton NJ, Kanani M, Roebuck DJ, et al. Tissue-Engineered Tracheal Replacement in a Child: A 4-Year Follow-Up Study. Am J Transplant 2015;15:2750-7. [Crossref] [PubMed]
- Elliott MJ, Butler CR, Varanou-Jenkins A, et al. Tracheal Replacement Therapy with a Stem Cell-Seeded Graft: Lessons from Compassionate Use Application of a GMP-Compliant Tissue-Engineered Medicine. Stem Cells Transl Med 2017;6:1458-64. [Crossref] [PubMed]