The genetic and molecular basis of bicuspid aortic valve associated thoracic aortopathy: a link to phenotype heterogeneity
Bicuspid aortic valve associated thoracic aortopathy (BAV-TA)
Bicuspid aortic valve (BAV) is the most common congenital cardiac anomaly, affecting 0.5-1.4% of the general population (1-4). BAV is a clinically heterogeneous disorder with a high rate of surgically relevant aortic valve and ascending aortic complications, which occur in over 35% of those affected (5). As such, BAV confers a greater burden of disease than all other congenital heart diseases combined (3,6). Familial clustering and genetic studies have established that BAV is a heritable trait, with approximately 9% prevalence amongst first-degree relatives, and up to 24% in families with more than one affected family member (3,7,8). Despite its importance, the etiology of BAV is largely undetermined, although it is likely to involve genetic heterogeneity, abnormal signaling pathways and aberrant neural crest cell migration (3). BAV is not only a disorder of valvular development, but also represents a complex coexistent genetic disease of the aorta and cardiac development (3,7).
Thoracic aortic enlargement is common in BAV, reported in up to 50-60% of affected individuals (6,9,10). Significant phenotype heterogeneity, which may occur independently of the underlying valvular morphology and function (6,9-11), has been described, most commonly manifesting as asymmetric ascending aortic dilatation beyond the sinotubular junction with variable arch involvement and/or varying degrees of annuloaortic ectasia, predisposing to aortic dissection/rupture, a feared complication. Indeed, BAV conveys an 8-fold increased risk of aortic dissection and over a 25-year period, the risk for aneurysm formation is 26% and for aortic surgery is 25% (2), further highlighting its health burden. Presently, ongoing controversies exist in the literature regarding the underlying pathogenesis of BAV-associated thoracic aortopathy (BAV-TA), specifically whether it is genetic or hemodynamic in origin, which have important implications for planning intervention. Intense work is currently ongoing to address this question, which has shed light on the pathogenesis of BAV-TA. Therefore, it is timely to review the current state of knowledge in this common association of BAV, focusing primarily on the interaction between genetics, molecular pathway and hemodynamic factors in influencing the heterogeneous manifestation of aortopathy seen in BAV patients.
Genes, flow and phenotype heterogeneity in BAV-TA
Genetic underpinnings of BAV-TA
The developmental nature of BAV-TA, which implies the presence of an underlying genetic defect causing inherent structural abnormality of the arterial wall, has been suggested by various studies. Aortic root and ascending aortic dilatation have been documented in children with BAV (12-14), suggesting that BAV-TA is a process that begins early in life. Progressive aortic dilatation can also occur in patients with normally functioning BAV and even following aortic valve replacement (12,15-18), implying the presence of an intrinsically ‘weaker’ aorta. In addition, the presence of valve dysfunction alone does not necessarily account for the degree or rate of progression of aortic dilatation in BAV patients (10,16,19), suggesting that factors, other than hemodynamics, are at play. Notably, an inherent structural alteration has been described in the ascending aorta of BAV patients, irrespective of the underlying valve hemodynamics (20). Histological examination of dilated aortas of BAV patients demonstrates accelerated aortic media degeneration with focal matrix disruption, altered collagen content, greater elastin fragmentation, accumulation of mucopolysaccharide ground substance and higher rates of vascular smooth muscle cell (VSMC) apoptosis (Figure 1) (16,21). Notably, these findings are also present in the non-dilated aortas of BAV patients and similar to those observed in the aortas of Marfan syndrome patients with Fibrillin-1 gene mutation (16,21,22). In fact, deficiency of fibrillin-1, which occurs independently of aortic diameter, and increased matrix metalloproteinase-2 (MMP2) activity have been reported in BAV aortic tissue (20). Furthermore, inherent abnormality of aortic elasticity is observed commonly in BAV patients, even at the non-dilated stage and irrespective of aortic size (23). It has thereby been hypothesized that loss of fibrillin-1 microfibrils in BAV aortas, presumably from an underlying heritable microfibrillar gene defect, may cause detachment of VSMC from the elastic laminae, resulting in accelerated cell death and the release of pathological MMP species with subsequent matrix degradation, weakening of aortic wall structural integrity, loss of aortic elasticity, progressive aortic dilatation, and aneurysm and/or dissection (20). Interestingly, fibrillin-1 deficiency is also observed in the pulmonary arteries of BAV patients and main pulmonary artery dilatation has been noted to occur in association with BAV in the absence of pulmonary valve abnormality (20,24). It is therefore speculated that both aortic and main pulmonary artery dilatation in BAV may be the result of a common developmental abnormality as the great arteries originate from a common embryonic trunk (20). Overall, these findings suggest an underlying genetic defect in the pathogenesis of aortic dilatation in BAV.
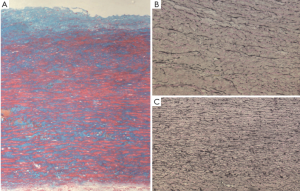
Hemodynamic contributions in BAV-TA
There is emerging evidence to support the hypothesis that BAV-TA is a hemodynamic phenomenon, in which the structurally defective valve, or even a ‘normally’ functioning one, is in fact morphologically stenotic, as a result of restricted mobility/opening of the conjoined leaflet, producing a nonaxial transvalvular turbulent flow jet within the aortic root (25-27). This creates abnormal biomechanics and helical flow alterations that propagate eccentrically inside the proximal aorta, resulting in uneven wall stress distribution and promoting aortic dilatation/aneurysm formation, which preferentially bulges asymmetrically toward the right-anterior aortic wall (i.e., aortic convexity) (28). A positive correlation has also been demonstrated between the degree of restriction in the conjoint cusp systolic motion (the so-called “conjoint cusp opening angle”) and the consequent eccentricity of the transvalvular systolic flow, with the severity and growth rate of the ascending aortic dilatation (27,29,30). Furthermore, in support of the hemodynamic theory, different aortic leaflet fusion patterns have been shown to generate specific jet orientations in the proximal aorta (28), with distinct patterns of asymmetry in the aneurysms that develop different rates of aortopathy progression, and unique MMPs and TIMPs (MMP inhibitors) signatures (31,32). Relatively recently, using sophisticated 4D flow cardiac MRI, fusion of the right and left coronary leaflets (R-L BAV), the most common type of BAV, has been shown to produce a right-anteriorly directed helical systolic flow jet with marked peripheral skewing towards the ascending aortic convexity (28). This may explain the larger aortic root dimension and asymmetric dilatation of the mid-ascending aorta, commonly seen in these BAV patients, and the more severe degree of ascending aortic wall degeneration with enhanced proteolytic indices relative to the other BAV subtypes (26,31,32). Meanwhile, fusion of the right and noncoronary leaflets (R-N BAV), the second most common type of BAV, is associated with left-posteriorly directed eccentric flow jet with propagation further towards the proximal aortic arch, which might explain its association with isolated ascending aortic dilatation (without aortic root involvement) and increased aortic arch dimensions seen in this subgroup of BAV patients (26,33).
Further support for the importance of flow in BAV-TA pathogenesis has also arisen from in vivo studies, which show that aberrant shear stress, such as that produced by BAV leaflets, is able to modulate expression of genes that regulate MMP production, matrix remodeling and VSMC apoptosis, thus predisposing to aneurysm formation (34,35). This is substantiated by the increased expression of MMP2 and a higher MMP2 to TIMP1 activity in tissue samples from aortic aneurysms in BAV patients (16), as well as a higher apoptosis rate of cultured VSMC derived from the dilated aorta of BAV patients compared to controls (21). Furthermore, BAV aortic aneurysm tissues exhibit reduction of endothelial nitric oxide synthase (eNOS) protein expression, with site-dependent variability, which is probably triggered by variations in shear stress along the aortic wall (15,36). Simulated reduction of eNOS expression in the cultured VSMCs of BAV-related aneurysm tissue, by treatment with NOS inhibitor L-NAME, results in cytosolic accumulation of HTRA2/Omi, a proapoptotic mitochondrial serine protease, which presumably mediates VSMC apoptosis in BAV aortas (36), thereby conferring susceptibility to aneurysm formation. Moreover, structural alteration of the extracellular matrix in itself can also result in transmission of proapoptotic cues to the VSMCs (37). Extracellular matrix remodelling occurs early in BAV, evident even in the nonaneurysmal BAV aortas, with maximum changes observed at the convexity, and is characterized by collagen and laminin reduction and increases in fibronectin and tenascin expression (37,38). These changes are associated with enhanced VSMC apoptotic indices, also primarily at the convexity, even prior to overt aortic dilatation, and also, interestingly, increased Bcl-2-modifying factor-Bcl-2 binding (Bmf-Bcl2), a known matrix-dependent proapoptotic signaling mechanism (37). Therefore, it is speculated that stress-induced extracellular matrix changes within the aortic convexity of BAV patients may trigger cytoskeletal rearrangement of VSMCs that lead to early local apoptosis of these cells. This is presumably mediated by Bmf-Bcl2 binding, resulting in progressive, typically asymmetric aortic enlargement seen in BAV-TA (37). These studies support the important role of hemodynamics in the initiation and progression of BAV-TA.
Phenotype heterogeneity in BAV-TA
Not all patients with BAV will develop aortic dilatation over time, regardless of the underlying valvular function. There is also a highly heterogenous expression of aortopathy in BAV disease even amongst patients with specific valve fusion pattern and similar levels of valve dysfunction (39). Furthermore, there is a small subset of BAV patients, mostly young males, who present with predominantly symmetric dilatation of the aortic root at an early age. This is associated with annular dilatation and varying degrees of aortic insufficiency (10) and may represent a predominantly genetic form of BAV disease less influenced by hemodynamic factors.
Therefore, current evidence suggests that BAV-TA is a product of the interaction between genetic and hemodynamic factors (Figure 2). BAV-TA could be associated with a congenital anomaly of some component of a matrix signalling pathway, thereby conferring inherent genetic susceptibility. Meanwhile, the hemodynamic disturbances, produced by the genetically determined, anatomically abnormal valve, then act after birth as a triggering and accelerating factor to the locally degenerative process within the aortic media, leading to progressive asymmetric aortic dilatation and aneurysm formation (37). Ultimately, the clinical heterogeneity seen in BAV-TA disease may be explained by individual variable combinations of coexisting genetic susceptibilities, the severity of hemodynamic alteration and other yet-to-be-identified environmental factors in modifying the final phenotype manifestation of the aortopathy.
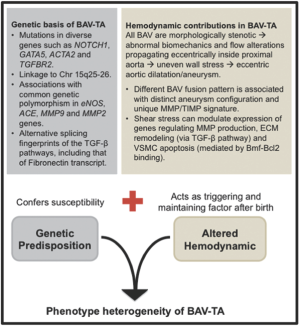
Genetic and molecular basis of BAV-TA: evidence for a divergent origin?
Genetic studies have established that BAV has an underlying genetic basis, although the causative genes linked to the defect remain largely unknown. Determining the genetics of BAV is complex and it is likely due to mutations in different genes (genetic heterogeneity) with variable patterns of inheritance (3). BAV is probably the result of mutations in diverse genes encoding transcription factors, extracellular matrix proteins and signaling pathways that regulate cell proliferation, differentiation, adhesion or apoptosis (40). To date, only the transcriptional regulator NOTCH1 gene at chromosome 9q34.3 has been linked to the development and progression of non-syndrome associated BAV in humans, in a limited number of familial cases and approximately 4% of sporadic cases (41,42). In animal studies, mice with homozygous deletion of the eNOS (Nos3) gene (43) or those with cardiac homeobox Nkx2-5 haploinsufficiency (44), and a certain strain of Syrian hamsters belonging to a family subjected to systematic inbreeding in Sans-Coma’s laboratory (45) have been associated with a higher incidence of BAV. However, individual mutations in these genes alone have not been associated with human cases of BAV and notably, nor have they been associated with thoracic aortic aneurysm development in these murine models (46,47). Meanwhile, targeted deletion of the cardiac transcription factor Gata5 in mice leads to partially penetrant BAV with R-N morphology (48). The essential role of GATA5 in cardiogenesis and aortic valve development has only recently been reported, whereby it regulates several pathways involved in endocardial cell differentiation, including those directed by Bmp4, Tbx20, and notably, NOS3 and NOTCH1 (49). As previously mentioned, mutations in the latter 2 genes have been respectively associated with BAV in mice and in humans.
It is possible that the genes responsible for BAV may also account for the development of the associated thoracic aortopathy, because of a shared embryological origin of the aortic valve and the ascending aorta from the cardiac neural crest cells (16). Indeed, NOTCH1 non-synonymous variants have been associated with coexisting cases of BAV and BAV-TA in approximately 4-10% of cases (42,50), although the molecular mechanisms by which NOTCH1 variants result in BAV and BAV-TA remain to be elucidated. Interestingly, a number of rare non-synonymous variations within the highly conserved and functionally important GATA5 transcriptional activation domains have recently been reported in up to 4% of sporadic cases of human BAV and its associated aortopathy (51). This finding provides support for the role of GATA5 as a potential candidate gene in modulating the pathogenesis of BAV/BAV-TA. Meanwhile, genome-wide marker-based linkage analyses have provided further evidence on the genetic basis of BAV-TA with some families with BAV and ascending aortic aneurysms showing linkage to chromosome 15q25-26 (52), although the causal gene(s) are yet to be identified.
Interestingly, different cases of BAV-TA may have their own unique pathogenetic basis (53). For example, BAV has been observed to occur more frequently in patients with thoracic aortic aneurysm who have mutations in the FBN1 and ACTA2 genes (54). The latter gene is located on chromosome 10q and encodes smooth muscle alpha-actin (ACTA2). A single nucleotide substitution in the TGFBR2 gene, c1159G>A, which results in an amino acid change (i.e. missense mutation) and destabilizes the mutant TGFBR2 protein structure of the aortic media, was segregated in a family with non-syndrome associated BAV and proximal aortic aneurysm (53), although the true incidence of TGFBR mutation in the overall BAV population is probably very low (55).
Common genetic variants could also play a role in the occurrence and progression of thoracic aortopathy (56). Associations have been reported between the risk of aneurysm formation in BAV patients with polymorphisms in eNOS, angiotensin-converting enzyme (ACE), MMP9 and MMP2 genes (56,57). Indeed, these genetic variants may become invaluable in clinical practice as genetic biomarkers for increased susceptibility to aortopathy development in BAV.
Recently, a comparative study on global gene expression levels was performed in aortic tissues from patients with bicuspid or tricuspid aortic valve, with and without thoracic aortic aneurysm (58). Only 7 genes showed differential expression in aortas from BAV patients without aortic dilatation and patients with a tricuspid aortic valve without aortic dilatation, providing further clues to the underlying pathogenetic basis of BAV disease. The 4 upregulated genes were LEFTY2 (a TGF-β family member), FRAS1 (a member of extracellular matrix family of protein), SHC4 and DAPK3 (a proapoptotic gene), and the 3 downregulated genes were VEGFC (a member of vascular endothelial growth factor family), NFASC (a member of the L1 family of cell adhesion molecules) and LSP1 (lymphocyte-specific protein-1). Given the possible shared genetic underpinnings of BAV and the related aortopathy, investigation of these genes in BAV/BAV-TA is warranted (47). The study also showed general overexpression of immune-related genes in the dilated aortas of tricuspid aortic valve patients compared with the dilated aortas of BAV patients, suggesting that immune response genes are not directly involved in BAV-TA (58). Rather, BAV patients with aortic dilatation showed an almost exclusive expression of the TGF-β binding proteins LTBP3/4, ADAMTSL1 and an alternatively spliced isoform of Fibronectin-1 (FN1) (58,59). A common extracellular matrix component of the vessel wall, fibronectin is a glycoprotein that influences cell migration and proliferation, thus central to normal tissue repair/remodeling and maintenance of tissue integrity in response to injury. Patients with BAV-TA appear to have impaired TGF-β-mediated splicing mechanisms of fibronectin transcript, thereby leading to altered fibronectin expression in the aortic aneurysm tissue and consequently contributing to the increased susceptibility to aortopathy development in BAV patients (59). Interestingly, a diverging alternative splicing fingerprint of the TGF-β pathways has been observed within ascending aortic dilatation in bicuspid versus tricuspid aortic valve patients (60), resulting in differential downstream gene expression and signaling. Different epigenetic modification of the chromatin structure may be responsible for the observed differential splicing pattern of TGF-β (60), which may play an important contributory role in modifying phenotypic heterogeneity of thoracic aneurysm pattern in BAV. Importantly, these findings highlight the central role of TGF-β as the key regulator of vascular matrix remodeling and VSMC activity, and that dysregulation of TGF-β signaling and its downstream pathways appears to be a common mechanism involved in aneurysm formation, including in BAV-TA. TGF-β signaling is extremely complex, and intense research to unravel these pathways is currently ongoing.
Interestingly, evidence is emerging to suggest that the genetic basis of BAV-TA may be distinct from that of the underlying valve defect. Several studies have demonstrated that ascending thoracic aortopathy is common (~30%) among first-degree relatives of BAV patients, including those with a normal tricuspid aortic valve, and impairment of aortic root elastic properties have also been observed in these individuals (61,62). More recently, an echocardiography-based study performed in families enriched for BAV demonstrated that both aortic and pulmonary artery diameters are quantitative traits that exhibit significant heritability, and BAV acts as an independent modifying factor, which influences the dimensions of the great arteries (63). Therefore, these studies suggest that BAV and the ‘associated aortopathy’ might in fact have distinct genetic etiologies (63), although flow pattern alterations created by the abnormal leaflets may contribute towards aortopathy development in BAV patients. This finding is important as it suggests that family members of BAV patients may also be at risk for thoracic aneurysm, even if they do not have BAV (63), and this highlights the importance of family screening. Intriguingly, this raises the question whether BAV is a primarily heritable valvular disorder with aortopathy as an associated secondary phenomenon, whether the aberrant leaflets simply represent a “clinical marker” for an underlying primary heritable vascular disorder, or whether BAV and its associated aortopathy have an oligogenic inheritance pattern, where at least 2 co-segregated genetic mutations are required for disease development. Further studies to elucidate these uncertainties are warranted.
Future directions
Current evidence supports a genetic basis for BAV and the associated aortopathy, although the causative genes remain largely undetermined in the majority of cases. For BAV-TA, evidence is also growing to support the importance of altered hemodynamics in its pathogenesis, both as a triggering and a perpetuating factor. Indeed, genetic susceptibilities and hemodynamic influences are not mutually exclusive in their contributory role in BAV-TA pathogenesis. Rather, BAV-TA is likely to be the result of a complex interplay between specific gene mutations with their modifying loci, hemodynamic influences produced by the aberrant leaflets and stochastic factors (47). The balance of this interaction is likely to have a major impact on the ultimate disease expression and the phenotype heterogeneity seen in BAV-TA. The main challenge faced by clinicians today lies in the limited ability to determine preoperatively the leading factor, either hemodynamic or genetic, that plays the predominant role in disease development in each individual case of BAV-TA. Cases in which genetic factor plays the dominant role may be associated with a more aggressive disease phenotype that has a tendency toward dissection, and thus may justify earlier and more extensive aortic intervention. Therefore, there is a clear and urgent need to improve our ability to risk stratify the different forms of BAV-TA, to distinguish the less versus the more “malignant” forms of aortopathy. Recent advances in cardiac MRI techniques, using sophisticated 4D flow assessments and measurements of conjoint cusp opening angles, as well as identification of various peripheral biomarkers and genetic polymorphisms, which mark aneurysm susceptibility, have represented important steps towards an improved and individually tailored management of BAV-related aortopathy. Further validation of these clinical and biological/genetic markers in a larger, well defined BAV cohort and development of future diagnostic and risk stratification tools in BAV-TA are awaited with great interest.
Acknowledgements
RP is supported by a co-funded Postgraduate Scholarship (PC 10S 5530) from the National Health and Medical Research Council (NHMRC) and the National Heart Foundation of Australia (NHFA), Australia. CS is the recipient of a National Health and Medical Research Council (NHMRC) of Australia Practitioner Fellowship. The authors acknowledge the support from the Cardiology, Cardiothoracic and Anatomical Pathology Department of the Royal Prince Alfred Hospital, Australia. Special thanks to Dr Geoff Watson for providing histological images used in the manuscript.
Disclosure: The authors declare no conflict of interest.
References
- Basso C, Boschello M, Perrone C, et al. An echocardiographic survey of primary school children for bicuspid aortic valve. Am J Cardiol 2004;93:661-3.
- Michelena HI, Khanna AD, Mahoney D, et al. Incidence of aortic complications in patients with bicuspid aortic valves. JAMA 2011;306:1104-12.
- Siu SC, Silversides CK. Bicuspid aortic valve disease. J Am Coll Cardiol 2010;55:2789-800.
- Tutar E, Ekici F, Atalay S, et al. The prevalence of bicuspid aortic valve in newborns by echocardiographic screening. Am Heart J 2005;150:513-5.
- Vallely MP, Semsarian C, Bannon PG. Management of the ascending aorta in patients with bicuspid aortic valve disease. Heart Lung Circ 2008;17:357-63.
- Ward C. Clinical significance of the bicuspid aortic valve. Heart 2000;83:81-5.
- Cripe L, Andelfinger G, Martin LJ, et al. Bicuspid aortic valve is heritable. J Am Coll Cardiol 2004;44:138-43.
- Huntington K, Hunter AG, Chan KL. A prospective study to assess the frequency of familial clustering of congenital bicuspid aortic valve. J Am Coll Cardiol 1997;30:1809-12.
- Tzemos N, Therrien J, Yip J, et al. Outcomes in adults with bicuspid aortic valves. JAMA 2008;300:1317-25.
- Nistri S, Sorbo MD, Marin M, et al. Aortic root dilatation in young men with normally functioning bicuspid aortic valves. Heart 1999;82:19-22.
- Jackson V, Petrini J, Caidahl K, et al. Bicuspid aortic valve leaflet morphology in relation to aortic root morphology: a study of 300 patients undergoing open-heart surgery. Eur J Cardiothorac Surg 2011;40:e118-24.
- Gurvitz M, Chang RK, Drant S, et al. Frequency of aortic root dilation in children with a bicuspid aortic valve. Am J Cardiol 2004;94:1337-40.
- Holmes KW, Lehmann CU, Dalal D, et al. Progressive dilation of the ascending aorta in children with isolated bicuspid aortic valve. Am J Cardiol 2007;99:978-83.
- Fernandes S, Khairy P, Graham DA, et al. Bicuspid aortic valve and associated aortic dilation in the young. Heart 2012;98:1014-9.
- Aicher D, Urbich C, Zeiher A, et al. Endothelial nitric oxide synthase in bicuspid aortic valve disease. Ann Thorac Surg 2007;83:1290-4.
- Tadros TM, Klein MD, Shapira OM. Ascending aortic dilatation associated with bicuspid aortic valve: pathophysiology, molecular biology, and clinical implications. Circulation 2009;119:880-90.
- Michelena HI, Desjardins VA, Avierinos JF, et al. Natural history of asymptomatic patients with normally functioning or minimally dysfunctional bicuspid aortic valve in the community. Circulation 2008;117:2776-84.
- Yasuda H, Nakatani S, Stugaard M, Failure to prevent progressive dilation of ascending aorta by aortic valve replacement in patients with bicuspid aortic valve: comparison with tricuspid aortic valve. Circulation 2003;108:II291-4.
- Keane MG, Wiegers SE, Plappert T, et al. Bicuspid aortic valves are associated with aortic dilatation out of proportion to coexistent valvular lesions. Circulation 2000;102:III35-9.
- Fedak PW, de Sa MP, Verma S, et al. Vascular matrix remodeling in patients with bicuspid aortic valve malformations: implications for aortic dilatation. J Thorac Cardiovasc Surg 2003;126:797-806.
- Bonderman D, Gharehbaghi-Schnell E, Wollenek G, et al. Mechanisms underlying aortic dilatation in congenital aortic valve malformation. Circulation 1999;99:2138-43.
- Blunder S, Messner B, Aschacher T, et al. Characteristics of TAV- and BAV-associated thoracic aortic aneurysms--smooth muscle cell biology, expression profiling, and histological analyses. Atherosclerosis 2012;220:355-61.
- Nistri S, Grande-Allen J, Noale M, et al. Aortic elasticity and size in bicuspid aortic valve syndrome. Eur Heart J 2008;29:472-9.
- Kutty S, Kaul S, Danford CJ, et al. Main pulmonary artery dilation in association with congenital bicuspid aortic valve in the absence of pulmonary valve abnormality. Heart 2010;96:1756-61.
- Robicsek F, Thubrikar MJ, Cook JW, et al. The congenitally bicuspid aortic valve: how does it function? Why does it fail? Ann Thorac Surg 2004;77:177-85.
- Girdauskas E, Disha K, Borger MA, et al. Relation of bicuspid aortic valve morphology to the dilatation pattern of the proximal aorta: focus on the transvalvular flow. Cardiol Res Pract 2012;2012:478259.
- Della Corte A, Bancone C, Conti CA, et al. Restricted cusp motion in right-left type of bicuspid aortic valves: a new risk marker for aortopathy. J Thorac Cardiovasc Surg 2012;144:360-9, 369.e1.
- Hope MD, Hope TA, Crook SE, et al. 4D flow CMR in assessment of valve-related ascending aortic disease. JACC Cardiovasc Imaging 2011;4:781-7.
- den Reijer PM, Sallee D 3rd, van der Velden P, et al. Hemodynamic predictors of aortic dilatation in bicuspid aortic valve by velocity-encoded cardiovascular magnetic resonance. J Cardiovasc Magn Reson 2010;12:4.
- Hope MD, Wrenn J, Sigovan M, et al. Imaging biomarkers of aortic disease: increased growth rates with eccentric systolic flow. J Am Coll Cardiol 2012;60:356-7.
- Ikonomidis JS, Ruddy JM, Benton SM Jr, et al. Aortic dilatation with bicuspid aortic valves: cusp fusion correlates to matrix metalloproteinases and inhibitors. Ann Thorac Surg 2012;93:457-63.
- Russo CF, Cannata A, Lanfranconi M, et al. Is aortic wall degeneration related to bicuspid aortic valve anatomy in patients with valvular disease? J Thorac Cardiovasc Surg 2008;136:937-42.
- Fazel SS, Mallidi HR, Lee RS, et al. The aortopathy of bicuspid aortic valve disease has distinctive patterns and usually involves the transverse aortic arch. J Thorac Cardiovasc Surg 2008;135:901-7, 907.e1-2.
- Dolan JM, Meng H, Singh S, et al. High fluid shear stress and spatial shear stress gradients affect endothelial proliferation, survival, and alignment. Ann Biomed Eng 2011;39:1620-31.
- Ruddy JM, Jones JA, Stroud RE, et al. Differential effect of wall tension on matrix metalloproteinase promoter activation in the thoracic aorta. J Surg Res 2010;160:333-9.
- Mohamed SA, Radtke A, Saraei R, et al. Locally different endothelial nitric oxide synthase protein levels in ascending aortic aneurysms of bicuspid and tricuspid aortic valve. Cardiol Res Pract 2012;2012:165957.
- Della Corte A, Quarto C, Bancone C, et al. Spatiotemporal patterns of smooth muscle cell changes in ascending aortic dilatation with bicuspid and tricuspid aortic valve stenosis: focus on cell-matrix signaling. J Thorac Cardiovasc Surg 2008;135:8-18, 18.e1-2.
- Della Corte A, De Santo LS, Montagnani S, et al. Spatial patterns of matrix protein expression in dilated ascending aorta with aortic regurgitation: congenital bicuspid valve versus Marfan’s syndrome. J Heart Valve Dis 2006;15:20-7; discussion 27.
- Barker AJ, Markl M, Bürk J, et al. Bicuspid aortic valve is associated with altered wall shear stress in the ascending aorta. Circ Cardiovasc Imaging 2012;5:457-66.
- Martin LJ, Ramachandran V, Cripe LH, et al. Evidence in favor of linkage to human chromosomal regions 18q, 5q and 13q for bicuspid aortic valve and associated cardiovascular malformations. Hum Genet 2007;121:275-84.
- Garg V, Muth AN, Ransom JF, et al. Mutations in NOTCH1 cause aortic valve disease. Nature 2005;437:270-4.
- Mohamed SA, Aherrahrou Z, Liptau H, et al. Novel missense mutations (p.T596M and p.P1797H) in NOTCH1 in patients with bicuspid aortic valve. Biochem Biophys Res Commun 2006;345:1460-5.
- Lee TC, Zhao YD, Courtman DW, et al. Abnormal aortic valve development in mice lacking endothelial nitric oxide synthase. Circulation 2000;101:2345-8.
- Biben C, Weber R, Kesteven S, et al. Cardiac septal and valvular dysmorphogenesis in mice heterozygous for mutations in the homeobox gene Nkx2-5. Circ Res 2000;87:888-95.
- Fernández B, Durán AC, Fernández-Gallego T, et al. Bicuspid aortic valves with different spatial orientations of the leaflets are distinct etiological entities. J Am Coll Cardiol 2009;54:2312-8.
- Majumdar R, Yagubyan M, Sarkar G, et al. Bicuspid aortic valve and ascending aortic aneurysm are not associated with germline or somatic homeobox NKX2-5 gene polymorphism in 19 patients. J Thorac Cardiovasc Surg 2006;131:1301-5.
- Padang R, Bagnall RD, Semsarian C. Genetic basis of familial valvular heart disease. Circ Cardiovasc Genet 2012;5:569-80.
- Laforest B, Andelfinger G, Nemer M. Loss of Gata5 in mice leads to bicuspid aortic valve. J Clin Invest 2011;121:2876-87.
- Laforest B, Nemer M. Genetic insights into bicuspid aortic valve formation. Cardiol Res Pract 2012;2012:180297.
- McKellar SH, Tester DJ, Yagubyan M, et al. Novel NOTCH1 mutations in patients with bicuspid aortic valve disease and thoracic aortic aneurysms. J Thorac Cardiovasc Surg 2007;134:290-6.
- Padang R, Bagnall RD, Richmond DR, et al. Rare non-synonymous variations in the transcriptional activation domains of GATA5 in bicuspid aortic valve disease. J Mol Cell Cardiol 2012;53:277-81.
- Goh D, Han L, Judge D, et al. Linkage of familial bicuspid aortic valve with aortic aneurysm to chromosome 15q. Am J Hum Genet 2002;71:211.
- Girdauskas E, Schulz S, Borger MA, et al. Transforming growth factor-beta receptor type II mutation in a patient with bicuspid aortic valve disease and intraoperative aortic dissection. Ann Thorac Surg 2011;91:e70-1.
- Jondeau G, Boileau C. Genetics of thoracic aortic aneurysms. Curr Atheroscler Rep 2012;14:219-26.
- Arrington CB, Sower CT, Chuckwuk N, et al. Absence of TGFBR1 and TGFBR2 mutations in patients with bicuspid aortic valve and aortic dilation. Am J Cardiol 2008;102:629-31.
- Foffa I, Murzi M, Mariani M, et al. Angiotensin-converting enzyme insertion/deletion polymorphism is a risk factor for thoracic aortic aneurysm in patients with bicuspid or tricuspid aortic valves. J Thorac Cardiovasc Surg 2012;144:390-5.
- Pisano C, Maresi E, Balistreri CR, et al. Histological and genetic studies in patients with bicuspid aortic valve and ascending aorta complications. Interact Cardiovasc Thorac Surg 2012;14:300-6.
- Folkersen L, Wågsäter D, Paloschi V, et al. Unraveling divergent gene expression profiles in bicuspid and tricuspid aortic valve patients with thoracic aortic dilatation: the ASAP study. Mol Med 2011;17:1365-73.
- Paloschi V, Kurtovic S, Folkersen L, et al. Impaired splicing of fibronectin is associated with thoracic aortic aneurysm formation in patients with bicuspid aortic valve. Arterioscler Thromb Vasc Biol 2011;31:691-7.
- Kurtovic S, Paloschi V, Folkersen L, et al. Diverging alternative splicing fingerprints in the transforming growth factor-β signaling pathway identified in thoracic aortic aneurysms. Mol Med 2011;17:665-75.
- Loscalzo ML, Goh DL, Loeys B, et al. Familial thoracic aortic dilation and bicommissural aortic valve: a prospective analysis of natural history and inheritance. Am J Med Genet A 2007;143A:1960-7.
- Biner S, Rafique AM, Ray I, et al. Aortopathy is prevalent in relatives of bicuspid aortic valve patients. J Am Coll Cardiol 2009;53:2288-95.
- Martin LJ, Hinton RB, Zhang X, et al. Aorta Measurements are Heritable and Influenced by Bicuspid Aortic Valve. Front Genet 2011;2:61.