Radical pleurectomy and photodynamic therapy for malignant pleural mesothelioma
Surgery and malignant pleural mesothelioma
Malignant pleural mesothelioma (MPM) remains one of the most virulent cancers afflicting mankind. Pemetrexed-based chemotherapy is generally considered the standard of care for this disease, with surgery still relegated to investigational status. Furthermore, given that the cancer will almost always recur, surgery is technically a palliative procedure. That said, however, there is compelling evidence and general agreement within the MPM treatment community that surgery-based multimodal therapies offer some patients the best chance for a survival measured in years instead of months or weeks. Just who those patients are, what operation they should have and what elements should comprise the multimodal treatment are topics of much debate. That surgery is currently the only modality that can render a patient with no evidence of disease for this massive cancer, which typically exceeds hundreds of milliliters in volume, is not a topic of debate. That innovative approaches are needed to complement surgery is also not a topic of debate.
The most accepted concept, arguably the tenet of surgery-based treatments, is that the role of surgery is to achieve a macroscopic complete resection - that is, no visible or palpable disease remaining after the surgical debulking. In addition, there is general agreement that all surgery-based treatments should include pemetrexed-based chemotherapy, but the timing and duration of that therapy is highly variable. Beyond these two convictions, there is little agreement on current surgery-based treatment approaches. The two principal areas of controversy are the type of operation to perform and what additional treatments, if any, should be combined with surgery and chemotherapy. The operations for MPM can be broadly classified as lung-sacrificing and lung-sparing, with both approaches having relative merits and disadvantages.
Lung-sacrificing surgery most commonly takes the form of extrapleural pneumonectomy (EPP), arguably the accepted approach for lung-sacrificing surgery for MPM. The operation entails en bloc resection of mediastinal/bony hemithoracic parietal pleura, diaphragm, pericardium and lung, with subsequent prosthetic reconstruction of the diaphragm and pericardium. This operation has the advantages of: standard nomenclature, standard surgical approach, the ability to employ hemithoracic radiation as an adjuvant treatment and, almost certainly, leaving behind the lowest burden of microscopic disease. A less obvious advantage is that the operative plan is established preoperatively. That is, the patient and the healthcare team all know what to expect before, during and after the operation. That is not the case for many surgeons who perform lung-sparing surgery who, instead, predicate the choice of operation on intraoperative criteria such as the bulk of tumor or the degree of invasion of the cancer into the pulmonary fissures.
The principal disadvantage of EPP is that it leaves the patient with one lung. This extends beyond the expected compromise in quality of life during the patient’s period of remission in that, if the patient is less robust by virtue of having one lung, it can also compromise the types of treatments for which the patient is a candidate when they have their inevitable recurrence. As essentially all patients will recur, the level of aggressiveness in the treatments they are able to tolerate for that recurrence can impact their survival. Finally, another potential disadvantage of EPP is that the physiologic impact of pneumonectomy may preclude some patients from undergoing surgery-based treatments whereas they might be candidates for lung-sparing surgery.
Lung-sparing surgery has a multitude of disadvantages, starting with the fact that even the nomenclature is highly variable. Although there has been a motion to standardize the name of the operation as “pleurectomy-decortication”, numerous surgeons who perform lung-sparing surgery (including the author) take issue with this name as “decortication” implies preservation of the visceral pleura, which is typically resected in its entirety by this author as well as others who contest the suggested nomenclature (1). The term adopted by this and some other surgeons to describe the operation we perform is “radical pleurectomy”. Radical pleurectomy more accurately describes the operation, which entails resection of all pleural surfaces with preservation of the lung and, whenever possible, the phrenic nerve and partial thickness of the diaphragm and pericardium. Obviously, in light of the fact that even the nomenclature is variable, there is tremendous variability in both the perceived indications for lung-sparing surgery as well as the actual technique.
Some surgeons relegate lung-sparing surgery to patients with the earliest stages, essentially no detectable disease, while others utilize it for a “palliative debulking” for advanced stage disease and intentionally leave behind residual gross tumor. As stated previously even many proponents of lung-sparing surgery often decide upon that approach intraoperatively, based upon variable criteria. This engenders the disadvantage that nobody, neither the patient nor the surgeon, goes into the operation knowing what to expect. Anecdotally, some patients report this to be extremely stressful. Another disadvantage of lung-sparing surgery is that it makes it difficult or impossible to effectively employ hemithoracic radiation as an adjuvant therapy. This, combined with the fact that lung-sparing surgery almost certainly leaves behind more microscopic disease than EPP, are the likely reasons that some of the best local control results have been reported with protocols that include EPP and radiation (2). Finally, at least in this author’s hands, radical pleurectomy is a more challenging and time consuming operation than EPP.
Part of this, at least as the operation exists in its current iteration, is that it is more like an artistic than an engineering endeavor in that judgment is required to know “when it’s done”. That is, radical pleurectomy is more like painting a painting and EPP is more like building a bridge, in that EPP yields a single complete specimen that clearly declares the mission is accomplished. Radical pleurectomy, at least as we perform it, leaves behind multiple tumor-normal tissue interfaces that may require judgment, sometimes frozen section analyses, to declare a macroscopic complete resection has been achieved.
The advantage of lung-sparing surgery, however, is that it leaves the patients with two lungs. This typically translates into lower operative mortality, a greater pool of potential surgical candidates, better postoperative pulmonary function/quality of life than EPP and, perhaps, greater ability to tolerate adjuvant therapies for the inevitable recurrence. The potential survival advantage of this last point cannot be underestimated as essentially all MPM patients will recur after surgery and their treatment options at that point can significantly affect their outcomes.
Thus, there are relative merits to both surgical approaches and it remains to be established which approach is optimal and in which settings. Whereas adjuvant external beam radiation is typically limited to EPP, just like adjuvant/neoadjuvant systemic therapy can be combined with either surgical approach, both can be combined with intraoperative adjuvant therapies. The current intraoperative adjuvants in use include: hyperthermic chemotherapy, hyperthermic povidone iodine and photodynamic therapy (PDT).
Photodynamic therapy (PDT)
PDT is a light-based cancer treatment where the patient receives a nontoxic photosensitizing compound that is subsequently activated with visible light. Skeptics of the ability to combine visible light and nontoxic compounds to produce powerful chemical reactions need look no further than chlorophyll and sunlight for the process of photosynthesis, without which life on earth as we know it would not exist. Most photosensitizers are cyclic structures, tetrapyrroles, that are derived from porphyrins, chlorins or bacteriochlorins.
When these substances capture energy from light photons, in the presence of oxygen, triplet oxygen is produced. Energy transfer can then occur, resulting in the production of singlet oxygen. Singlet oxygen has a half-life in the microsecond range, is highly reactive and is thought to be the initial trigger in producing the multitude of PDT mechanisms. Because singlet oxygen appears to be the principle effector of PDT, oxygen is generally considered an essential component for PDT, along with light and photosensitizer. There is speculation, however, that other mechanisms may occur which are oxygen independent and allow for direct transfer of energy from the activated photosensitizer to other recipients, like water (3,4).
Some of the known mechanisms of PDT include direct cell killing, selective destruction of neovasculature and initiation of a tumor-directed immune response (5-7). Which modes of action come into play and which dominate are highly variable and incompletely understood. The different modes are known to be affected by: the photosensitizer, the target tissue, the route of photosensitizer administration, the dose of photosensitizer, the timing of photosensitizer administration, local oxygen availability, the amount of light given, the wavelength of light given and even the rate at which the light is given (8,9). For example, the same dose of photosensitizer being activated by the same dose of light at the same wavelength may act primarily by a vascular mechanism with a short drug-light interval, direct cell kill at a longer drug-light interval or induced apoptosis at the same drug-light interval but with the light delivered at a lower fluence rate. PDT is truly an extraordinarily interesting, customizable and flexible treatment modality. With respect to the application of PDT for MPM, we consider the tumor-direct immune effect as the most intriguing and, potentially, the most exploitable for improving current surgery-based treatment of MPM and for developing future treatments for MPM.
PDT is unusual, perhaps because it works by a number of different mechanisms, in that it is one of the only cancer treatments, along with surgery, that has potential to be curative with a single treatment. It is not thought to have, like radiation or some chemotherapeutics, a cumulative toxicity. Thus, although not typically applicable for MPM, it presents the option of being administered repeatedly.
As any impactful treatment for MPM must be multimodal, PDT is well suited as an adjuvant in that it is compatible with essentially all treatment modalities and potentially additive or synergistic with some such as: immunotherapies, targeted therapies, hyperthermia and metabolic-targeted therapies (10-13). In addition to the therapeutic compatibility of PDT with current MPM treatment modalities, it has another aspect that is intuitively appealing as an intraoperative adjuvant - depth of penetration.
Because the visible light used for pleural PDT penetrates tissue for several millimeters, the PDT treatment effect extends for several millimeters beneath the surface. It is, therefore, conceptually a well-suited intraoperative complement to an operation that predictably leaves behind microscopic cancer at the surface. Furthermore, the fact that this effect extends for only several millimeters, as opposed to much deeper or full thickness like radiation, it is conceptually an ideal treatment to combine with lung-sparing surgery where the goal is to penetrate the “nooks and crannies” at the surface of the denuded lung but not damage any appreciable volume of the functional lung parenchyma.
Combining photodynamic therapy with surgery for malignant pleural mesothelioma
Prior to surgery the patient receives photosensitizer as an outpatient, immediately becomes light sensitive and, therefore, requires preinjection education regarding exposure to sunlight and intense incandescent lighting before and after surgery. Over the years we have learned that with proper patient education complications from, and even complaints about, cutaneous photosensitivity have become a nonexistent.
Our current surgery-based approach to MPM is to combine pemetrexed-based chemotherapy, intraoperative PDT and lung-sparing surgery. Currently we exclusively perform lung-sparing surgery, radical pleurectomy, to achieve a macroscopic complete resection. Whether or not this is the best operation for MPM and whether or not there are instances where an EPP would be a better operation are unanswered questions at this time and not the focus of this discourse. In our hands, however, this is currently the approach yielding the best results with this specific treatment combination. To be clear, radical pleurectomy is performed in every case, regardless of whether or not the patient would tolerate a pneumonectomy and regardless of the extent of the cancer. In more than a 100 of these cases, many with extensive fissure invasion and many with large bulk tumors (Figure S1), there has not been an instance where removing the lung would have contributed to the ability to achieve a macroscopic complete resection. Our radical pleurectomy procedure distills down to mobilizing the cancer, such that it is tethered solely to the lung, and then removing the entire visceral pleura en bloc with the cancer - preserving the lung and as much of the diaphragm, pericardium and phrenic nerve as possible.
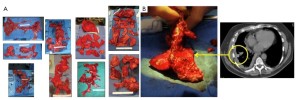
The photosensitive patient does require light precautions to be taken during surgery. This includes making the incision with only the fluorescent room lights on and then shielding the skin prior to turning on headlamps and overhead surgical lights. Yellow filters are also used to limit unmeasured light administration, as the photosensitizers absorb less in the yellow region of visible light (Figure 1). The operation commences identical to an EPP by separating the cancer from the bony hemithorax. It is then separated from the pericardium and diaphragm, typically performing split thickness resections (Figure 2). At this point the cancer is tethered to the lung, which is then inflated on a separate ventilation circuit and the visceral pleura is removed from the lung, along with the cancer (Figure 3). Removal of cancers extending into the pulmonary fissures may require skeletonization of the pulmonary artery (Figure 4). This is not a contraindication to radical pleurectomy, does not mandate conversion to a pneumonectomy and can be reliably accomplished with even extensive involvement of the fissure. Depending upon the consistency of the cancer, sometimes the specimen must be removed piecemeal and sometimes it will come out as unified specimen (Figure S1A). Large tumor bulk greater than a liter, even two liters, is not a contraindication to radical pleurectomy and does not necessitate conversion to a pneumonectomy. Once the cancer has been removed, then a macroscopic complete resection has been achieved (Figure S2). A thoracic lymphadenectomy is then performed, harvesting nodes from all named stations in the hemithorax. In addition, the author has started to harvest the posterior intercostal lymph nodes, accessed by incision into the endothoracic fascia at the level of the rib heads. The significance of these nodes, not part of any current staging system, is unclear but our group has some preliminary data suggesting they may have prognostic significance.
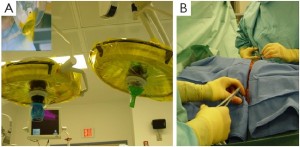

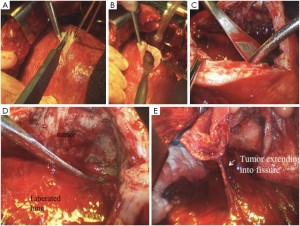
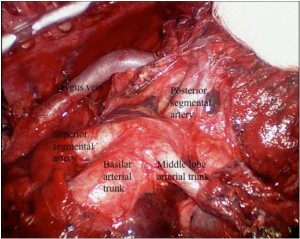
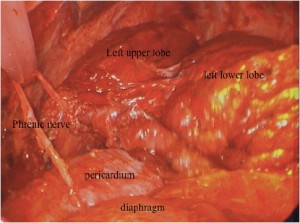
Once a macroscopic complete resection is achieved, the intraoperative PDT is performed and usually takes about an hour to complete. The first step in intraoperative PDT is to sew in isotropic light detectors into strategic locations within the chest cavity. These detectors are then fed into a dosimetry device that provides real time feedback on both current fluence rate of light and the total dose of light at the specified wavelength that has been recorded by that detector (Figure S3).
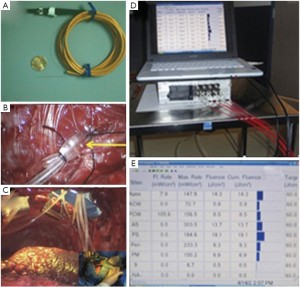
A laser supplies light of the appropriate wavelength. At one time the lasers for PDT were of essentially prohibitive cost, but now the light for intraoperative PDT can be supplied with a portable briefcase-sized diode laser, replacing the wheeled steamer trunk-sized dye module lasers that preceded them and the immobile optical table-based lasers that preceded them. The laser fiber is placed into a modified endotracheal tube, where the balloon is filled with intralipid solution. This helps disperse the light 360 degrees and also protects the patient from the fiber tip, which can get very hot. Dilute intralipid is also poured into the chest cavity to help reflect the light into all recesses and to avoid pooling of blood which would shield the underlying tissue from the light. The PDT is accomplished by moving the light source around the chest until each of the light detectors registers the predetermined light dose (Figure 5).
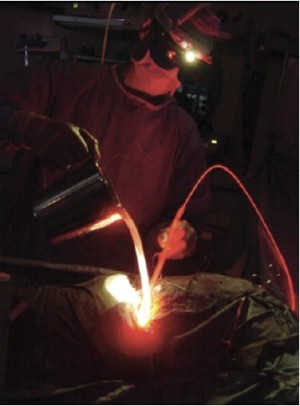
The treatment is empiric; it would not be possible to calculate or plan delivery for the light due to the complex reflective and refractive geometry within the chest cavity. Performing the PDT in this manner, by measuring the light, allows it to be done safely.
Results combining surgery and intraoperative PDT for MPM
Our first PDT-surgery study for MPM was a Phase I study with a new photosensitizer (14). The results were encouraging enough that we were planning a Phase II study, but the drug became unavailable and we were forced to use a photosensitizer, porfimer sodium, which had not been shown to make a difference in a previous Phase III study comparing surgery and immunochemotherapy +/- PDT (15). As a result we started our series by performing EPP with intraoperative porfirmer sodium PDT, and with the intention of following with both pemetrexed-based chemotherapy and hemithoracic radiation. Over time, however, with an eye toward attempting to preserve quality of life and being able to offer an aggressive treatment option to patients who were not appropriate candidates for pneumonectomy, we switched to lung-sparing surgery. After we had completed an equal number of each operation, 14 in each group, we did a comparative analysis (16). Although not randomized, the two patient cohorts were surprisingly well matched and despite our quality of life motivation, we found a significant survival advantage in the radical pleurectomy group (Figure 6). It was based upon this pilot study that our group switched to our current protocol of exclusively performing lung-sparing surgery.
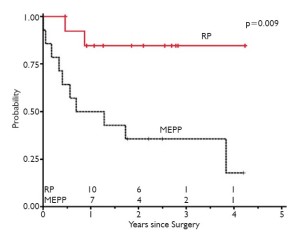
We subsequently reported on a series of 38 patients who underwent radical pleurectomy and intraoperative PDT, of whom 97% had Stage III or IV disease and 18% had nonepithelial histology (17). The overall median survival from the time of surgery was 31.7 months. When separated by histology, the most prognostic factor (P<0.001), the 31 patients with epithelial disease had an overall survival of 41.2 months while the 7 patients with nonepithelial histology had an overall survival of only 6.8 months. The 20 epithelial patients with N2 disease had an overall survival of 31.7 months while the 11 patients without N2 disease had a median survival of 57.1 months (Figures 7-10, Table 1).
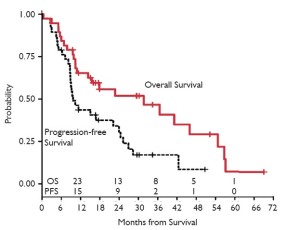
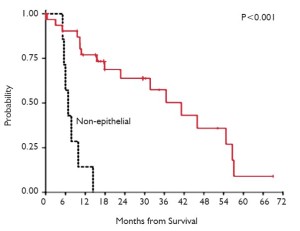
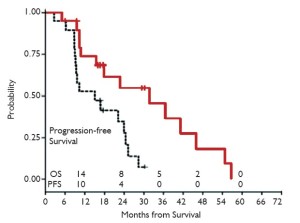
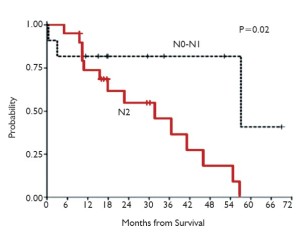
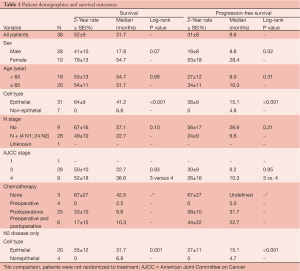
Full table
These survivals were calculated from the time of surgery, not diagnosis or first treatment as is sometimes reported and which can significantly extend the survival time. There was a 3% operative mortality and a macroscopic complete resection was achieved in 97% of the cases. Complications were typically what would be expected with this type of surgery, with a persistent air leak in approximately 10% of the patients. The main postoperative complication attributable to PDT presented like an accelerated and transient case of ARDS and was managed with aggressive diuresis (Figure S4). At the median follow-up of 34.4 months, used for all analyses, there was an isolated local failure in 26% of the patients and simultaneous local and distant failure in another 40%, for a total local failure in two thirds of the patients.
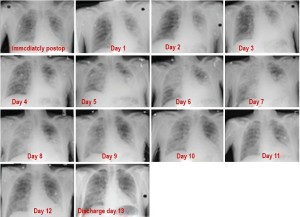
The limitations of this study are primarily those associated with any small nonrandomized retrospective study, in addition to the fact that preoperative and postoperative treatments were not completely standardized. Nonetheless, in a cohort of such advanced stage patients, the results are intriguing and the approach merits consideration.
Perhaps the most intriguing aspect of these results is the unusually long overall survival despite the high local failure rate of 66% (isolated local failure 26% combined with simultaneous local and distant failure 40%). The reasons for the prolonged survivals noted in our study, despite the high local recurrence rates, are unclear. Our current hypotheses include: having two lungs permits more adjuvant treatment options at the time of recurrence, having two lungs facilitates an overall more robust state of health and consequent longer survival and/or the remaining PDT-treated cancer cells induced an “autologous tumor vaccine” effect. Killing cancer cells with PDT has been shown to be one of the most effective ways to stimulate a tumor-directed immune response with an autologous tumor vaccine (18). Clearly all operations for malignant pleural mesothelioma, especially lung-sparing operations, leave behind cancer cells. The possibility exists, therefore, that these PDT-treated cells that are left behind may be inducing this type of vaccine effect. Exploring these hypotheses, especially the immune response to surgery-PDT, are all areas of active investigation by our group. In addition, these results have inspired translational research geared toward developing combined surgery-PDT-immunotherapy treatments as well as developing a PDT-generated autologous tumor vaccine that could be used as an adjuvant or de novo treatment.
Conclusions
PDT is a unique cancer treatment that works by a multitude of mechanisms and has intuitive and theoretical appeal as an intraoperative adjuvant for surgery-based MPM multimodal protocols. It can be combined with surgery in a safe manner by measuring the dose of light that is given. Incorporating PDT into our surgery-based treatment scheme, we performed a pilot study that indicated our patients were better served with lung-sparing surgery and subsequently obtained results on a larger cohort that yielded some of the most promising data that has been reported for MPM. Whether or not PDT is stimulating a tumor-directed immune response in these patients and whether or not the PDT is even playing a role in the extended survival of these patients are each the focus of active investigation by our group. In addition to immunologic studies, particularly with respect to T-cell response to the tumors before and after PDT, we are planning a prospective randomized trial for radical pleurectomy and pemetrexed-based chemotherapy, plus-minus intraoperative PDT. This trial should establish whether or not our current use of PDT is playing a positive role in the treatment of our patients. Regardless, the known powerful immunologic effects produced by PDT are of considerable interest and may be exploitable for the development of new treatments for MPM.
Acknowledgements
Disclosure: The author has no conflict of interest disclosure and received no help in preparation of the manuscript.
References
- Rice D, Rusch V, Pass H, et al. Recommendations for uniform definitions of surgical techniques for malignant pleural mesothelioma: a consensus report of the International Association for the Study of Lung Cancer International Staging Committee and the International Mesothelioma Interest Group. J Thorac Oncol 2011;6:1304-12.
- Rice DC, Stevens CW, Correa AM, et al. Outcomes after extrapleural pneumonectomy and intensity-modulated radiation therapy for malignant pleural mesothelioma. Ann Thorac Surg 2007;84:1685-92; discussion 1692-3.
- Allison RR, Sibata CH. Oncologic photodynamic therapy photosensitizers: a clinical review. Photodiagnosis Photodyn Ther 2010;7:61-75.
- Gorman A, Killoran J, O’Shea C, et al. In vitro demonstration of the heavy-atom effect for photodynamic therapy. J Am Chem Soc 2004;126:10619-31.
- Brackett CM, Gollnick SO. Photodynamic therapy enhancement of anti-tumor immunity. Photochem Photobiol Sci 2011;10:649-52.
- Castano AP, Mroz P, Hamblin MR. Photodynamic therapy and anti-tumour immunity. Nat Rev Cancer 2006;6:535-45.
- Mroz P, Hashmi JT, Huang YY, et al. Stimulation of anti-tumor immunity by photodynamic therapy. Expert Rev Clin Immunol 2011;7:75-91.
- Bisland SK, Lilge L, Lin A, et al. Metronomic photodynamic therapy as a new paradigm for photodynamic therapy: rationale and preclinical evaluation of technical feasibility for treating malignant brain tumors. Photochem Photobiol 2004;80:22-30.
- Wang HW, Rickter E, Yuan M, et al. Effect of photosensitizer dose on fluence rate responses to photodynamic therapy. Photochem Photobiol 2007;83:1040-8.
- Yanase S, Nomura J, Matsumura Y, et al. Synergistic increase in osteosarcoma cell sensitivity to photodynamic therapy with aminolevulinic acid hexyl ester in the presence of hyperthermia. Photomed Laser Surg 2009;27:791-7.
- Kwitniewski M, Juzeniene A, Glosnicka R, et al. Immunotherapy: a way to improve the therapeutic outcome of photodynamic therapy? Photochem Photobiol Sci 2008;7:1011-7.
- Kwitniewski M, Moan J, Juzeniene A. Metabolic-targeted therapy with dichloroacetate (DCA): a novel treatment strategy to improve the outcome of photodynamic therapy. Photochem Photobiol Sci 2011;10:25-8.
- Chio-Srichan S, Oudrhiri N, Bennaceur-Griscelli A, et al. Toxicity and phototoxicity of Hypocrellin A on malignant human cell lines, evidence of a synergistic action of photodynamic therapy with Imatinib mesylate. J Photochem Photobiol B 2010;99:100-4.
- Friedberg JS, Mick R, Stevenson J, et al. A phase I study of Foscan-mediated photodynamic therapy and surgery in patients with mesothelioma. Ann Thorac Surg 2003;75:952-9.
- Pass HI, Temeck BK, Kranda K, et al. Phase III randomized trial of surgery with or without intraoperative photodynamic therapy and postoperative immunochemotherapy for malignant pleural mesothelioma. Ann Surg Oncol 1997;4:628-33.
- Friedberg JS, Mick R, Culligan M, et al. Photodynamic therapy and the evolution of a lung-sparing surgical treatment for mesothelioma. Ann Thorac Surg 2011;91:1738-45.
- Friedberg JS, Culligan MJ, Mick R, et al. Radical pleurectomy and intraoperative photodynamic therapy for malignant pleural mesothelioma. Ann Thorac Surg 2012;93:1658-65; discussion 1665-7.
- Gollnick SO, Vaughan L, Henderson BW. Generation of effective antitumor vaccines using photodynamic therapy. Cancer Res 2002;62:1604-8.