Mesothelin-targeted immunotherapies for malignant pleural mesothelioma
Introduction
Despite the use of aggressive trimodality therapy consisting of neoadjuvant chemotherapy, surgery, and hemithoracic radiation, the median survival of patients with malignant pleural mesothelioma (MPM) is 12 to 18 months (1,2). Immune responses have been shown to be beneficial in MPM patients (3,4); therefore, focusing immunotherapeutic strategies on promoting these immune responses is an attractive approach. One such approach involves targeting cancer-associated antigens highly expressed on mesothelioma cells by use of monoclonal antibodies, recombinant immunotoxins, vaccines, or genetically engineered T cells. Targeted antigens can be either cell-surface antigens, such as mesothelin, or intracellular antigens, such as WT-1. Cell-surface antigens are favored for the immunotherapeutic approach because of their ease of targeting.
Mesothelin as a cancer-antigen target in MPM
Antigen expression
Mesothelin is a 70-KDa cell-surface glycoprotein expressed at very low levels by a single layer of mesothelial cells lining the pleura, peritoneum, and pericardium (5). High-level expression of surface mesothelin has been reported in multiple malignancies, including mesothelioma (5-8). While others have reported mesothelin expression in MPM (9), our group has documented uniform and high-level cell-surface expression of mesothelin in epitheloid and biphasic MPM, which was retained in tumor samples even after chemotherapy, as well as in metastatic lymph nodes (10).
Pathobiology
Studies suggest that mesothelin plays a role in tumorigenesis by increasing cellular proliferation and migration (11) and is a marker of neoplastic progression (12). Our group has demonstrated—both in an orthotopic MPM mouse model and in patients—that mesothelin contributes to the locoregional aggressiveness characteristic of MPM (10). Mesothelin overexpression is associated with the expression of metalloproteinase-9 (MMP-9), a protein involved in the degradation of extracellular matrix, facilitating cancer cell migration and local invasion (10).
Biomarker
Robinson et al. first reported that the determination of soluble mesothelin-related protein (SMRP) levels in the serum could be used as a marker for detection of MPM, with a sensitivity of 84% and a specificity of 100% (13). Preclinical and clinical studies have now demonstrated that serum SMRP levels correlate with stage and tumor burden and may be a useful marker for following the therapy response in patients with MPM (14,15).
Mesothelin-targeted immunotherapy
Given its high levels of expression in MPM and its limited expression in normal tissues, mesothelin provides a safe target for tumor-specific therapies. The following mesothelin-targeted immunotherapeutic approaches are currently the focus of clinical trials (Table 1).
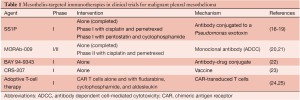
Full table
Recombinant immunotoxin
SS1P is an immunotoxin that comprises a high-affinity antimesothelin mouse antibody chemically conjugated to a Pseudomonas exotoxin (26). In preclinical models, SS1P has shown regression of mesothelin-expressing tumors, both alone and in conjunction with chemotherapy and radiation therapy (26-29). Evaluation of two phase I single-agent clinical trials (consisting of 58 mesothelin-expressing solid tumor patients) showed 5 subjects with partial response and 18 with stable disease. A dose-limiting toxicity in these trials was pleuritis, possibly caused by the targeting of normal mesothelial cells in the pleura (16,17). A third phase I trial, treating MPM patients with SS1P plus a standard regimen of chemotherapy, demonstrated 7 patients with partial response, 3 with stable disease, and 4 with progressive disease; the trial is now in the expansion phase (18).
Monoclonal antibody
MORAb-009 is a high-affinity chimeric monoclonal antibody that consists of a mouse antimesothelin single-chain variable fragment that binds mesothelin, grafted to a human IgG1 and κ constant regions (30). MORAb-009 works by eliciting antibody-dependent cellular cytotoxicity in which cells of the immune system lyse target cells expressing the mesothelin-bound antibody. In a phase I single-agent clinical study in which patients with refractory mesothelin-expressing solid tumors were treated, no complete or partial response was noted; 11 subjects had stable disease, and 9 had progressive disease. MORAb-009 displayed a promising safety profile, with the most common adverse reaction being infusion-related drug hypersensitivity that was amenable to antihistamine (20). Currently, phase II clinical trial of MORAb-009 in combination with chemotherapy is ongoing in mesothelioma patients (21).
Antibody-drug conjugates
Two mesothelin antibody-drug conjugates that have undergone preclinical testing are MDX-1382 (Medarex), an antibody conjugated to a potent DNA alkylating agent, and BAY 94-9343 (Bayer Healthcare), an antibody linked to a DM4 moiety that binds to tubulin (31,32). A phase I clinical trial of BAY 94-9343 for patients with solid tumors recently opened (22), and the drug has been adopted by the United States Orphan Drug Act, which will encourage its development and shorten the regulatory review and approval process for MPM patients.
Antimesothelin vaccine
CRS-207 is a vaccine that uses a live, attenuated strain of the bacterium Listeria monocytogenes to present human mesothelin to the host immune system (33). Recently, 17 patients (including 5 patients with mesothelioma) were treated in a phase I clinical trial (23). Although no objective tumor response was seen, CRS-207 was well-tolerated, and immune activation was observed, with 5 of 10 patients showing mesothelin-specific T-cell response. A phase II clinical trial in pancreatic cancer patients treated with CRS-207 and granulocyte macrophage-colony stimulating factor-expressing cells showed promising results, with prolonged survival observed in patients with mesothelin-specific immune cell responses (34).
Adoptive T-cell therapy
One recent advance in immunotherapy is the ability to use peripheral blood lymphocytes to generate genetically redirected T cells that target tumor antigens by the use of retroviral and lentiviral vectors (35). The safety and long-term persistence of these viral vectors were documented in a decade-long follow-up of HIV patients (36). These engineered T cells have the ability to circumvent tolerance to tumor antigens such as mesothelin (37). Furthermore, the ability to genetically reprogram T cells allows for the addition of costimulatory molecules, such as CD-28 and 4-1BB, to increase T-cell survival and expansion and to prevent anergy. In these cells, antigen recognition is usually achieved by expressing antigen receptors, which consist of either physiological, major histocompatibility complex (MHC)-restricted T cell receptors, or non-MHC-restricted chimeric antigen receptors (CARs) (35,38). Currently, there are two phase I clinical trials using antimesothelin CAR-transduced T cells. A National Cancer Institute clinical trial is treating metastatic or unresectable cancers that express mesothelin with CAR T cells, in conjunction with myeloablative chemotherapy and/or aldesleukin (an interleukin (IL)-2 analog), to augment CAR T-cell persistence (24). In the second phase I clinical trial, MPM patients are receiving 1 to 3 doses of mesothelin-targeting CAR T cells (25). In this trial, CARs are introduced into the T cells by electromicroporation, to enhance safety by limiting the survival of the T cells (39).
Current perspectives in mesothelin-targeted immunotherapy
Clinically relevant mouse model
The flank- and peritoneal-tumor animal models that have been used to date in preclinical studies of MPM do not reflect the clinical progression or outcomes seen in MPM patients. To recapitulate clinical MPM, our group has developed an orthotopic MPM mouse model (40). The resultant encasing tumors mimic human disease anatomically, with gross pleural and mediastinal tumor distribution and frequent chest wall and diaphragmatic invasion. In addition to metastasis to mediastinal lymph nodes, extensive angiogenesis and lymphogenesis attributable to high-level secretion of vascular endothelial growth factor were observed (10). Notably, tumor burden and progression can be reliably measured by magnetic resonance imaging, bioluminescence imaging, and serum SMRP levels (40). An orthotopic MPM mouse model that recapitulates human MPM pathobiology not only provides an appropriate in vivo tumor microenvironment in which to investigate novel therapeutic agents but also generates information regarding pharmacokinetics and tumor delivery of agents that will aid in designing future clinical trials.
Targeting tumor heterogeneity
Although epithelioid MPM expresses mesothelin uniformly, biphasic MPM tumors have a potential heterogeneity, which increases the complexity of targeting a single antigen. It is therefore critical to appropriately characterize the antigen expression of the targeted tumor, as well as that of other potentially exploitable targets. Targeting mesothelin-expressing cells may lead to a less-aggressive, more-manageable tumor that is possibly more susceptible to standard therapeutic agents, such as chemotherapy.
Targeted agent delivery to the tumor microenvironment
In clinical trials, shed mesothelin has been shown to cause low antitumor efficacy, because of binding of SS1P antibody to serum mesothelin, with a decreased amount of antibody reaching the tumor (16). A subsequent study found increased antitumor efficacy by combining taxol and SS1P, possibly attributable to taxol decreasing mesothelin shedding (41). Other strategies have focused on enhancing the tumor trafficking of the therapeutic agent by simultaneously targeting tumor-associated chemokines. Moon et al. added a chemokine receptor to a CAR, thereby increasing T-cell trafficking to the tumor (42).
On-target off-tumor toxicity
The potential targeting of normal cells expressing mesothelin may have been seen in the SS1P trial in which the dose-limiting toxicity was pleuritis. Therefore, strategies to protect against possible toxicities are needed. One approach to prevent adverse outcomes in CAR T-cell therapy is to incorporate a safety, or “suicide”, gene in the transferred cells. In this strategy, a prodrug that is administered for use in the case of an adverse event is activated by the suicide-gene product and kills the transduced cell (43). Three promising suicide genes—herpes simplex virus thymidine kinase (HSV-TK), deoxycytidine kinase (dCK), and inducible caspase 9 (44,45)—are currently undergoing investigation by our group. An added translational advantage of HSV-TK and dCK is their ability to be imaged by positron emission tomography scan, which may serve as a useful tool for future T-cell studies.
Host immune response
Immunogenicity to the SS1P immunotoxin may have inhibited maximal drug delivery to the tumor microenvironment; such a concern is the reason for pretreating patients with the lymphoablative drugs pentostatin and cyclophosphamide in a current clinical trial (19). In CAR T-cell therapy, immunomodulating cytokines, such as IL-12, have proven to be efficacious in decreasing the host immune response, thus decreasing the need for lymphoablation (46).
Conclusions
Mesothelioma-specific overexpression of mesothelin and its role in tumor aggressiveness provide an opportunity to target mesothelin with immunotherapeutic agents. The recent advances in the understanding of the kinetics of therapeutic-agent distribution and associated limiting factors, through noninvasive tumor and T-cell imaging, as well as the ability to measure serum SMRP levels, have enhanced our ability to obtain maximal information for clinical trials in rare diseases such as mesothelioma. Measures to counteract the immune-inhibitory environment, such as therapy with anti-PD1 or anti-CTLA-4, have not yet been investigated in MPM. This approach, which combines promoting an immune response with counteracting an immune-inhibitory environment, will play a significant role in the future course of immunotherapeutic treatment of MPM.
Acknowledgements
Disclosure: Work in the thoracic oncology and immunotherapy laboratory was supported in part by the Department of Defense (grants PR101053/LC110202); National Cancer Institute (grants CA164568/CA164585, U54CA137788/U54CA132378); Mesothelioma Applied Research Foundation (MARF) Grant in Memory of Lance S. Ruble; William H. Goodwin and Alice Goodwin, the Commonwealth Foundation for Cancer Research, the Experimental Therapeutics Center of Memorial Sloan-Kettering Cancer Center; American Association for Thoracic Surgery (AATS) - Third Edward D. Churchill Research Scholarship; International Association for the Study of Lung Cancer (IASLC) Young Investigator Award; and the National Lung Cancer Partnership/LUNGevity Foundation Research Grant.
References
- Krug LM, Pass HI, Rusch VW, et al. Multicenter phase II trial of neoadjuvant pemetrexed plus cisplatin followed by extrapleural pneumonectomy and radiation for malignant pleural mesothelioma. J Clin Oncol 2009;27:3007-13.
- Flores RM, Krug LM, Rosenzweig KE, et al. Induction chemotherapy, extrapleural pneumonectomy, and postoperative high-dose radiotherapy for locally advanced malignant pleural mesothelioma: a phase II trial. J Thorac Oncol 2006;1:289-95.
- Bograd AJ, Suzuki K, Vertes E, et al. Immune responses and immunotherapeutic interventions in malignant pleural mesothelioma. Cancer Immunol Immunother 2011;60:1509-27.
- Suzuki K, Kadota K, Sima CS, et al. Chronic inflammation in tumor stroma is an independent predictor of prolonged survival in epithelioid malignant pleural mesothelioma patients. Cancer Immunol Immunother 2011;60:1721-8.
- Chang K, Pastan I. Molecular cloning of mesothelin, a differentiation antigen present on mesothelium, mesotheliomas, and ovarian cancers. Proc Natl Acad Sci U S A 1996;93:136-40.
- Argani P, Iacobuzio-Donahue C, Ryu B, et al. Mesothelin is overexpressed in the vast majority of ductal adenocarcinomas of the pancreas: identification of a new pancreatic cancer marker by serial analysis of gene expression (SAGE). Clin Cancer Res 2001;7:3862-8.
- Ordóñez NG. The immunohistochemical diagnosis of mesothelioma: a comparative study of epithelioid mesothelioma and lung adenocarcinoma. Am J Surg Pathol 2003;27:1031-51.
- Tchou J, Wang LC, Selven B, et al. Mesothelin, a novel immunotherapy target for triple negative breast cancer. Breast Cancer Res Treat 2012;133:799-804.
- Chang K, Pai LH, Pass H, et al. Monoclonal antibody K1 reacts with epithelial mesothelioma but not with lung adenocarcinoma. Am J Surg Pathol 1992;16:259-68.
- Servais EL, Colovos C, Rodriguez L, et al. Mesothelin overexpression promotes mesothelioma cell invasion and MMP-9 secretion in an orthotopic mouse model and in epithelioid pleural mesothelioma patients. Clin Cancer Res 2012;18:2478-89.
- Li M, Bharadwaj U, Zhang R, et al. Mesothelin is a malignant factor and therapeutic vaccine target for pancreatic cancer. Mol Cancer Ther 2008;7:286-96.
- Rizk NP, Servais EL, Tang LH, et al. Tissue and serum mesothelin are potential markers of neoplastic progression in Barrett’s associated esophageal adenocarcinoma. Cancer Epidemiol Biomarkers Prev 2012;21:482-6.
- Robinson BW, Creaney J, Lake R, et al. Mesothelin-family proteins and diagnosis of mesothelioma. Lancet 2003;362:1612-6.
- Servais EL, Suzuki K, Colovos C, et al. An in vivo platform for tumor biomarker assessment. PLoS One 2011;6:e26722.
- Wheatley-Price P, Yang B, Patsios D, et al. Soluble mesothelin-related Peptide and osteopontin as markers of response in malignant mesothelioma. J Clin Oncol 2010;28:3316-22.
- Hassan R, Bullock S, Premkumar A, et al. Phase I study of SS1P, a recombinant anti-mesothelin immunotoxin given as a bolus I.V. infusion to patients with mesothelin-expressing mesothelioma, ovarian, and pancreatic cancers. Clin Cancer Res 2007;13:5144-9.
- Kreitman RJ, Hassan R, Fitzgerald DJ, et al. Phase I trial of continuous infusion anti-mesothelin recombinant immunotoxin SS1P. Clin Cancer Res 2009;15:5274-9.
- Kelly RJ, Sharon E, Pastan I, et al. Mesothelin-targeted agents in clinical trials and in preclinical development. Mol Cancer Ther 2012;11:517-25.
- Available online: http://clinicaltrials.gov NCT01362790
- Hassan R, Cohen SJ, Phillips M, et al. Phase I clinical trial of the chimeric anti-mesothelin monoclonal antibody MORAb-009 in patients with mesothelin-expressing cancers. Clin Cancer Res 2010;16:6132-8.
- Available online: http://clinicaltrials.gov NCT00738582
- Available online: http://ClinicalTrials.gov NCT01439152
- Le DT, Brockstedt DG, Nir-Paz R, et al. A live-attenuated Listeria vaccine (ANZ-100) and a live-attenuated Listeria vaccine expressing mesothelin (CRS-207) for advanced cancers: phase I studies of safety and immune induction. Clin Cancer Res 2012;18:858-68.
- Available online: http://ClinicalTrials.gov NCT01583686
- Available online: http://ClinicalTrials.gov NCT01355965
- Chowdhury PS, Viner JL, Beers R, et al. Isolation of a high-affinity stable single-chain Fv specific for mesothelin from DNA-immunized mice by phage display and construction of a recombinant immunotoxin with anti-tumor activity. Proc Natl Acad Sci U S A 1998;95:669-74.
- Mossoba ME, Onda M, Taylor J, et al. Pentostatin plus cyclophosphamide safely and effectively prevents immunotoxin immunogenicity in murine hosts. Clin Cancer Res 2011;17:3697-705.
- Hassan R, Broaddus VC, Wilson S, et al. Anti-mesothelin immunotoxin SS1P in combination with gemcitabine results in increased activity against mesothelin-expressing tumor xenografts. Clin Cancer Res 2007;13:7166-71.
- Hassan R, Williams-Gould J, Steinberg SM, et al. Tumor-directed radiation and the immunotoxin SS1P in the treatment of mesothelin-expressing tumor xenografts. Clin Cancer Res 2006;12:4983-8.
- Hassan R, Ebel W, Routhier EL, et al. Preclinical evaluation of MORAb-009, a chimeric antibody targeting tumor-associated mesothelin. Cancer Immun 2007;7:20.
- Rao C. Efficacy and toxicity of an anti-mesothelin antibody drug conjugate. 100th Annual Meeting of the American Association for Cancer Research 2009.
- Golfier S KA, Heisler I, Kopitz C, et al. Identification of BAY 94-9343, a mesothelin antibody-drug conjugate (ADC): characterization and anti-tumor activity in mesothelin positive preclinical tumor models. Proceedings of the 102nd Annual Meeting of the American Association for Cancer Research 2011.
- Brockstedt DG, Giedlin MA, Leong ML, et al. Listeria-based cancer vaccines that segregate immunogenicity from toxicity. Proc Natl Acad Sci U S A 2004;101:13832-7.
- Available online: http://ClinicalTrials.gov NCT01417000
- Sadelain M, Brentjens R, Rivière I. The promise and potential pitfalls of chimeric antigen receptors. Curr Opin Immunol 2009;21:215-23.
- Colovos C, Villena-Vargas J, Adusumilli PS. Safety and stability of retrovirally transduced chimeric antigen receptor T cells. Immunotherapy 2012;4:899-902.
- Sadelain M, Rivière I, Brentjens R. Targeting tumours with genetically enhanced T lymphocytes. Nat Rev Cancer 2003;3:35-45.
- Carpenito C, Milone MC, Hassan R, et al. Control of large, established tumor xenografts with genetically retargeted human T cells containing CD28 and CD137 domains. Proc Natl Acad Sci U S A 2009;106:3360-5.
- Zhao Y, Moon E, Carpenito C, et al. Multiple injections of electroporated autologous T cells expressing a chimeric antigen receptor mediate regression of human disseminated tumor. Cancer Res 2010;70:9053-61.
- Servais EL, Colovos C, Kachala SS, et al. Pre-clinical mouse models of primary and metastatic pleural cancers of the lung and breast and the use of bioluminescent imaging to monitor pleural tumor burden. Curr Protoc Pharmacol 2011;Chapter 14:Unit14.21.
- Zhang Y, Xiang L, Hassan R, et al. Immunotoxin and Taxol synergy results from a decrease in shed mesothelin levels in the extracellular space of tumors. Proc Natl Acad Sci U S A 2007;104:17099-104.
- Moon EK, Carpenito C, Sun J, et al. Expression of a functional CCR2 receptor enhances tumor localization and tumor eradication by retargeted human T cells expressing a mesothelin-specific chimeric antibody receptor. Clin Cancer Res 2011;17:4719-30.
- Bonini C, Ferrari G, Verzeletti S, et al. HSV-TK gene transfer into donor lymphocytes for control of allogeneic graft-versus-leukemia. Science 1997;276:1719-24.
- Likar Y, Zurita J, Dobrenkov K, et al. A new pyrimidine-specific reporter gene: a mutated human doxycytidine kinase suitable for PET during treatment with acycloguanosine-based cytotoxic drugs. J Nucl Med 2010;51:1395-403.
- Di Stasi A, Tey SK, Dotti G, et al. Inducible apoptosis as a safety switch for adoptive cell therapy. N Engl J Med 2011;365:1673-83.
- Pegram HJ, Lee JC, Hayman EG, et al. Tumor-targeted T cells modified to secrete IL-12 eradicate systemic tumors without need for prior conditioning. Blood 2012;119:4133-41.