Standard transthoracic echocardiography and transesophageal echocardiography views of mitral pathology that every surgeon should know
Introduction
Mitral valve disease is one of the most common valvular pathologies. As the population ages, the prevalence of significant mitral valve disease is increasing and expected to rise exponentially (1). Accordingly, the number of patients undergoing mitral valve surgery is also increasing (2). Echocardiography has become invaluable in the clinical management of mitral valve disease in the context of pre-surgical and post-surgical assessment. It is a non-invasive, readily accessible tool, which yields accurate information about the functional anatomy of the mitral valve and mechanisms of mitral valve disease. Systematic echocardiographic assessment of the various anatomical components of the mitral valve also provides information which guides decision-making regarding the timing and type of intervention needed. Finally, a number of echocardiographic parameters have been identified as predictors of the likely success of surgical intervention (3), which provides valuable prognostic information in regard to surgical outcomes. This enables an appropriate pre-operative assessment of risk. The aim of this review is to outline some of the standard echocardiographic features, which may be relevant to the surgical management of patients with mitral valve disease.
Overview of mitral valve anatomy
The mitral valve can essentially be divided into three main anatomical components: (I) annulus; (II) leaflets (anterior and posterior); and (III) subvalvular structures (chordae tendineae and papillary muscles). The mitral annulus is part of the fibrous skeleton of the heart and is composed of a fibromuscular ring situated between the left atrium and ventricle. The mitral valve leaflets attach to this annulus. The normal annulus is elliptical in shape, with an annular area between 5-11 cm2 (mean, 7 cm2), and has a saddle-shaped configuration (4-6). The annulus can be divided into anterior and posterior segments based on insertion of the corresponding anterior and posterior leaflets. It undergoes dynamic changes in shape during the cardiac cycle. It increases in size at the end of systole and reaches its maximum dimension at the end of diastole (7), while becoming less circular in systole than in diastole (5). The dynamic change in the size and shape of the mitral annulus is significant as it facilitates the opening motion and coaptation of the leaflets. Annular contraction occurs during systole resulting in narrowing of the valve’s orifice, which encourages leaflet apposition in combination with the apical force on commissural areas by the papillary muscles (8). The anterior part of the annulus is, however, relatively immobile compared to the posterior annulus. Hence, dynamic changes in the mitral annulus are likely due to movement of the posterior annulus. Pathological annular dilatation predominantly arises due to an increase in posterior annular circumference. This increases the anteroposterior valve dimension, thereby pulling the leaflets apart and compromising leaflet apposition (8).
The anterior and posterior leaflets of the mitral valve are similar in composition and thickness (approximately 1 mm) but have subtle morphological and functional differences. Both leaflets are essentially anchored to the annulus at their base. They have two distinct zones: the membranous zone, which forms the base of the leaflets and has a smooth and translucent appearance, and the coaptation zone, where the numerous chordae tendineae fuse. The coaptation zone is the rougher, more nodular and thicker portion of the leaflet (see Figure 1). The anterior leaflet is trapezoidal in shape, devoid of any well-defined indentations, extends vertically and is taller in height than the posterior leaflet. Via its attachment to one third of the circumference of the annulus, the anterior leaflet is also anchored to the fibrous portion of the annulus, which is more resistant to pathologic dilatation. As the cardiac fibrous skeleton becomes discontinuous away from the trigones along the mitral annulus, the posterior leaflet does not insert directly into the fibrous portion of the annulus. Instead, it inserts 2 mm from the fibrous portion of the annulus via a very thin band of connective tissue, predisposing this segment to dilatation and calcification (9). The posterior leaflet also typically has two identifiable clefts, which allow the leaflet to open fully during diastole. These clefts are used as anatomical landmarks to divide the posterior leaflet into three distinct segments, identified as P1, P2 and P3 (10). As the anterior leaflet is devoid of any prominent clefts, its division into the three segments A1, A2 and A3 is arbitrary and based on the adjacent segments of the posterior leaflet. The middle segment of the posterior leaflet, P2, typically has variable thickness and greater redundancy with the impact of greater systolic pressure, hence is more prone to prolapse and lesions (11,12). The distinct areas at which the annular attachments of the anterior and posterior leaflets come together are defined as the commissures and may represent a few millimeters of tissue or a well-developed leaflet segment. During systole, the margins of the anterior and posterior leaflets oppose over several millimeters at the coaptation zone. This zone is significant as its length can be a critical determinant of valve competency under several physiologic conditions. Normal mitral leaflets on two-dimensional echocardiography have the appearance of thin, translucent and highly mobile structures, which demonstrate maximum mobility at the leaflet tips (see Figure 2). Overall, the anterior mitral leaflet typically exhibits greater mobility in a number of views.
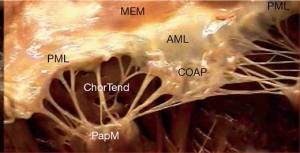
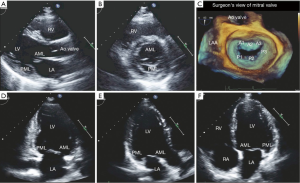
The subvalvular apparatus can be divided into the chordae tendineae and the papillary muscles. The chordae tendineae are filament-like structures of connective fibrous tissue that join the ventricular surface and free border of the leaflets to the fibrous heads of the papillary muscles and the posterior wall of the left ventricle. These form the leaflet suspension system that ultimately determines the position and tension on the leaflets at the end of systole. Marginal or “primary” chordae insert on the free margin of the leaflets and serve to prevent marginal prolapse and to align the coaptation zone. Intermediate or “secondary” chordae insert onto the ventricular face of the leaflets. These prevent the valve leaflet from developing excess tension and thus billowing by distributing tension across the ventricular surface of the leaflets. In addition, they may be important in maintaining dynamic ventricular shape and function due to their contribution to ventricular-valve continuity (13,14). Finally the basal or “tertiary” chordae are only associated with the posterior leaflet and connect the base of the leaflet and the posterior mitral annulus to the papillary muscles (Figure 3).
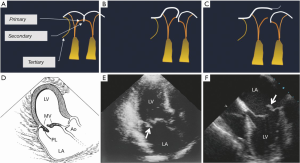
The papillary muscles arise from the area between the apical and middle thirds of the left ventricular free wall and are divided into two groups: the anterolateral and the posteromedial papillary muscles (15). The anterolateral papillary muscle is the larger of the two and has a single body but two heads (anterior and posterior heads). It is supplied by the first obtuse marginal branch of the circumflex artery and the first diagonal branch of the anterior descending artery. On the other hand, the posteromedial papillary muscle is smaller in size, has two bodies and three heads (anterior, intermediate and posterior heads). It is supplied by the posterior descending artery, a branch of the right coronary artery in 90% of cases and of the circumflex artery in the other 10% when it is much more vulnerable to ischemia (16). The attachment of the papillary muscles to the lateral wall of the left ventricle also means that the ventricle itself is an important functional component of the mitral valve. Hence any change in ventricular geometry that affects position of either papillary muscle can change the axial relationship of the chordae and leaflets and result in poor coaptation (8).
Mitral valve pathology and the key echocardiographic features
Due to the complex nature of mitral valve anatomy, coordination between all its independent anatomical components is required in order for the valve to function normally. Hence the echocardiographic assessment of the mitral valve should include a systematic segmental evaluation of its individual anatomical components as well as the valve as an entire unit in order to precisely identify specific pathologic abnormalities in valve structure and function. This requires integration of information obtained from both transthoracic and transesophageal echocardiography (TEE). Views and measurements enabled by transthoracic echocardiography (TTE), such as the mitral valve orifice area, and Doppler imaging, have been validated for a range of mitral valve pathologies. TEE is generally regarded as the standard of care for the surgical assessment of mitral valve disease. Currently, a comprehensive echocardiographic assessment should integrate information from multiple two-dimensional multi-planar tomographic views with and without color Doppler and three-dimensional imaging, if available. Echocardiography is also utilized to assess the success of a surgical procedure immediately post-operatively and for subsequent follow-up.
Mitral valve dysfunction can arise from pathology at any one of the multiple anatomical levels. Essentially, mitral valve pathology requiring surgical intervention can be broadly classified into either mitral regurgitation (MR) or mitral stenosis (MS). MR is by far more common in developed countries. Functional MR occurs in up to 50% of patients who present with systolic heart failure. The most common type of degenerative mitral valve disease is mitral valve prolapse, which occurs in 1-3% of the population in the United States. Conversely, rheumatic MS is the least common pathology affecting the mitral valve in the United States, accounting for less than 1% of cardiac diagnoses, although more frequently seen in developing nations (17).
Mitral stenosis (MS)
The two most common etiologies of MS are rheumatic MS and degenerative (calcific) MS. The main mechanism of rheumatic MS is commissural fusion. Other anatomic lesions such as chordal shortening, chordal fusion, leaflet thickening and superimposed calcification may restrict leaflet motion by causing progressive narrowing of the mitral valve orifice at the level of the leaflet tips. Key echocardiographic features include valve thickening (typically the posterior leaflet is thickened and restricted), restricted valve opening, anterior leaflet doming and fusion of the leaflets at the commissures. Additionally, leaflet thickening in rheumatic MS is typically most pronounced at the tips with relative sparing of the mid portion, which gives rise to the characteristic “hockey-stick” appearance of the leaflets. These changes are best appreciated on two-dimensional echocardiography in the parasternal long and short views as well as the apical views (see Figure 4A,B). M-mode imaging, which is typically performed in the parasternal long and short axis views, demonstrates a distortion of the pattern to one that is distinct for rheumatic MS, which involves anterior movement of the posterior valve in parallel to the anterior leaflet rather than a posterior motion (see Figure 4C). Real time three-dimensional echocardiography also allows visualization of the mitral valve en face and can be performed via transthoracic or TEE (see Figure 4D,E). Assessment of stenosis severity and the presence of complications of MS should also be performed. There are five validated methods for the assessment of severity of MS but the most common methods include measurement of the mitral orifice area by direct planimetry (see Figure 5) and assessment of gradients across the mitral valve by Doppler imaging or by the pressure half-time method (see Figure 6). Planimetry of the mitral valve is the current gold standard for the assessment of mitral valve orifice area as it is based on direct visualization of the mitral valve orifice and not limited by hemodynamic loading conditions. It has been shown to have excellent correlation with direct sizing at surgery but requires care so that planimetry is performed at the level of the leaflet tips (see Figure 5) (18,19). The Doppler-derived mean transmitral gradient is easily measured and is a useful tool to quantify severity. It is also reproducible and correlates well with invasive measurements. The gradient is assessed by the application of the simplified Bernoulli equation: ΔPressure = 4v2, where v is the transmitral velocity obtained from continuous-wave Doppler interrogation of the mitral flow in the 4-chamber view. This view allows for parallel alignment of the ultrasound beam and mitral inflow. The mean gradient is obtained from the average of digitized instantaneous gradients enveloped in the continuous-wave Doppler profile. Presently, integrated software automatically calculates and displays the peak and mean gradients (Figure 6A). Color Doppler is also useful in identifying eccentric diastolic mitral jets. These are often encountered in cases of severe deformity of the valvular and subvalvular apparatus and assist in guiding the placement of the Doppler beam through the highest flow velocity zone (18).
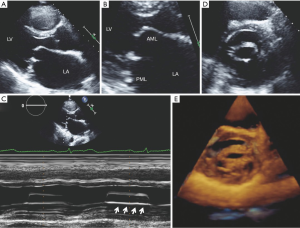
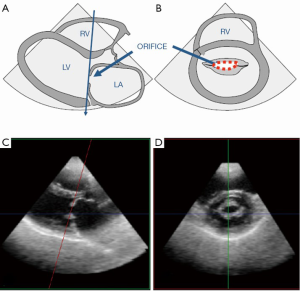
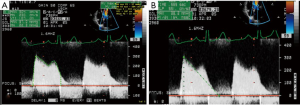
The appearance of calcific MS differs markedly from rheumatic MS. Calcific MS is commonly seen in association with hypertension, atherosclerotic disease, increased age and chronic kidney disease. In the case of non-rheumatic MS, there may be leaflet thickening and restriction of leaflet mobility but the commissures are rarely fused. Calcific MS results from annular calcification, which becomes heavy and encroaches onto the valve leaflets, resulting in thickening and/or calcification of the leaflets and a decrease in the anatomic orifice area. Calcification can also extend into the myocardium of the left ventricle and beneath the endocardial surface of the posterior leaflet (20). In calcific MS, the smallest mitral orifice area is at the base of the mitral leaflets rather than at the leaflet tips such as in rheumatic MS. On two-dimensional echocardiography, mitral annular calcification appears as echogenic material within the annular region and is best visualized on the parasternal long and short axis views (Figure 7). Apart from the morphological changes, the methods for evaluating stenosis severity are also different since many of the 2D and Doppler-derived techniques used for the assessment of gradients across the mitral valve cannot be reliably applied to calcific MS. This is due to the pattern of valve deformation and coexisting hemodynamic abnormalities. Planimetry of the mitral orifice area is difficult to perform in calcific MS since stenosis originates from heavy annular calcification that extends to the leaflets. Hence, the limiting orifice cannot be readily appreciated in short axis (21-23).
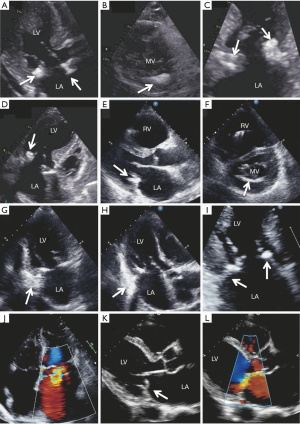
Mitral regurgitation (MR)
MR is the most common valvular disease with moderate or severe MR found in 1.7% of the general population, 6.4% of patients aged 65-74 years and 9.3% of those >75 years (1,24). Rheumatic heart disease is the most frequent cause of MR in developing countries, however degenerative or myxomatous mitral valve disease is the leading cause of MR in developed countries. Other disease entities such as ischemic heart disease and valvular damage from bacterial endocarditis also contribute as significant causes of MR (25). The approach to the assessment of MR needs to include assessment of valve dysfunction, lesions and the mechanism underlying the pathology, which is important for surgical decision-making. Although there are a number of classification schemes for MR, one commonly used scheme is that proposed by Alain Carpentier, which is based on the closing motion of the leaflets. It comprises: (I) type I dysfunction, which involves normal leaflet motion but severe annular dilatation resulting in a central regurgitant jet or perforation of one of the leaflets; (II) type II dysfunction, encompassing excessive leaflet motion secondary to pathologic elongation or rupture of the chordae tendineae and prolapse of the leaflet or a segment of the leaflet resulting in a regurgitant jet that is directed to the opposite side of the affected leaflet; and (III) type III dysfunction, which involves restricted leaflet motion due to retraction of the subvalvular apparatus. Two subtypes of type III dysfunction are possible: type IIIa, which is apical displacement of the leaflets and restricted leaflet motion during diastole and systole (tethering of the valve), frequently seen in rheumatic disease, inflammatory processes or papillary muscle displacement (from ischemic remodeling or dilated cardiomyopathy); and type IIIb, which is restricted leaflet motion predominantly during systole resulting in a regurgitant jet typically directed to the same side as the affected leaflet (10).
Mitral valve prolapse is primarily diagnosed by echocardiography and is defined as the systolic displacement of one or both mitral leaflets into the left atrium of greater than 2 mm (Figure 8A). It can typically occur with or without MR and in patients with evidence of leaflet thickening on echocardiography and myxomatous changes found on pathoanatomy. Degenerative disease of the mitral valve is typically a result of one of two opposing clinical entities: fibroelastic deficiency (FD) and Barlow’s disease (BD). Unfortunately, it is difficult to distinguish between these entities. Differentiation between the two is crucial as lesions due to BD are complex and frequently require expert surgical skill compared lesions due to FD, which are easier to repair (26-28). FD is the more common of the two and results from loss of mechanical integrity due to abnormalities of connective tissue structure or function. This typically results in localized or unisegmental prolapse or a flail leaflet (Figure 3). It is typically seen in patients in their sixth decade with a short, acute history of MR, likely due to rupture of a single mitral chord (26,29). On the other hand, BD results from an abnormal accumulation of mucopolysaccharides in the leaflets and chordae. This results in thick, bulky, redundant billowing leaflets and elongated chordae, which in turn results in prolapse of the leaflet(s) (Figure 8). This condition is typical in a younger female demographic and is usually relatively stable until the fourth decade (26,29). In regard to echocardiographic features, the key findings in BD are leaflets that have diffuse, complex lesions with prolapse and myxomatous degeneration of many segments in one or both leaflets. This may be due to excessive leaflet tissue, leaflet thickening, distension, elongation and thickening and/or the rupture of several chordae tendineae. These patients with BD also frequently have a dilated annulus and various degrees of annular and subvalvular apparatus calcification, particularly at the posterior face of the annulus and the posteromedial papillary muscle (30,31). This is in contrast to other conditions that also cause MR such as rheumatic disease, infective endocarditis or FD (8,32-34). Patients with FD typically have chordal rupture due to the progressive weakening and elongation of the chordae tendineae, usually involving the mid-segment of the posterior leaflet (Figure 3). The leaflets and the segments are usually completely normal with no change in height, size or tissue properties (35-38). However, it should be noted that chronic FD can occasionally result in distension of the prolapsing segment and demonstration of myxomatous characteristics (39). The key to distinguishing FD from other entities is exhaustive analysis of segments contiguous to the one that has prolapsed (40).
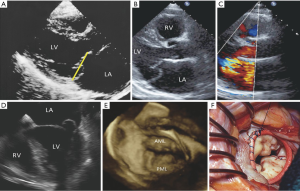
Ischemic heart disease is currently responsible for approximately 20% of patients with MR. MR due to ischemic heart disease can arise as a direct complication of a myocardial infarct, e.g., due to papillary muscle dysfunction or rupture, or as a consequence of left ventricular remodeling secondary to a myocardial infarct (41). Remodeling of the left ventricle results in apical and inferior displacement of the papillary muscles and tethering of the mitral leaflets, failure of coaptation and hence MR (Figure 9) (42,43). This is of particular significance in patients who have had a posterior infarction since remodeling may result in an asymmetric restriction of the posterior leaflet in systole (44,45). In contrast, patients with a dilated cardiomyopathy or anterior and posterior infarctions typically have both leaflets restricted (46).
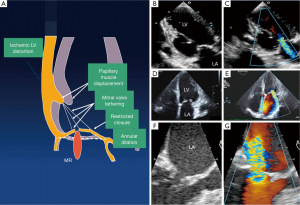
Conclusions
In conclusion, echocardiography plays an important role in the diagnosis and assessment of mitral valve pathology in the context of surgical management. Key echocardiographic features associated with particular disease entities enable the use of echocardiographic aids in the diagnosis, follow-up and subsequent clinical management of patients with mitral valve disease. The information obtained from a thorough echocardiographic assessment plays a significant role in the clinical decision-making process as to whether there should be surgical intervention and, if so, the necessary expertise needed. The availability of newer echocardiographic technology such as three-dimensional echocardiography has also improved our assessment of complex mitral valve lesions, allowing for improved pre-operative risk stratification. Precise follow-up of changes in geometry, volumes and hemodynamics is possible and this facilitates appropriate surgical intervention for patients.
Acknowledgements
None.
Footnote
Conflicts of Interest: The authors have no conflicts of interest to declare.
References
- Nkomo VT, Gardin JM, Skelton TN, et al. Burden of valvular heart diseases: a population-based study. Lancet 2006;368:1005-11. [PubMed]
- Gammie JS, Sheng S, Griffith BP, et al. Trends in mitral valve surgery in the United States: results from the Society of Thoracic Surgeons Adult Cardiac Surgery Database. Ann Thorac Surg 2009;87:1431-7; discussion 1437-9. [PubMed]
- Omran AS, Woo A, David TE, et al. Intraoperative transesophageal echocardiography accurately predicts mitral valve anatomy and suitability for repair. J Am Soc Echocardiogr 2002;15:950-7. [PubMed]
- Kopuz C, Erk K, Baris YS, et al. Morphometry of the fibrous ring of the mitral valve. Ann Anat 1995;177:151-4. [PubMed]
- Levine RA, Handschumacher MD, Sanfilippo AJ, et al. Three-dimensional echocardiographic reconstruction of the mitral valve, with implications for the diagnosis of mitral valve prolapse. Circulation 1989;80:589-98. [PubMed]
- Levine RA, Triulzi MO, Harrigan P, et al. The relationship of mitral annular shape to the diagnosis of mitral valve prolapse. Circulation 1987;75:756-67. [PubMed]
- Levine RA. Dynamic mitral regurgitation--more than meets the eye. N Engl J Med 2004;351:1681-4. [PubMed]
- Adams DH, Anyanwu AC, Sugeng L, et al. Degenerative mitral valve regurgitation: surgical echocardiography. Curr Cardiol Rep 2008;10:226-32. [PubMed]
- Nguyen TC, Itoh A, Carlhäll CJ, et al. The effect of pure mitral regurgitation on mitral annular geometry and three-dimensional saddle shape. J Thorac Cardiovasc Surg 2008;136:557-65. [PubMed]
- Carpentier A. Cardiac valve surgery--the "French correction". J Thorac Cardiovasc Surg 1983;86:323-37. [PubMed]
- Okamoto H, Itoh Y, Nara Y. Geometric analysis of the anterior mitral leaflet and mitral valve orifice in cadaveric hearts. Circ J 2007;71:1794-9. [PubMed]
- Pomerance A. Ballooning deformity (mucoid degeneration) of atrioventricular valves. Br Heart J 1969;31:343-51. [PubMed]
- Rodriguez F, Langer F, Harrington KB, et al. Importance of mitral valve second-order chordae for left ventricular geometry, wall thickening mechanics, and global systolic function. Circulation 2004;110:II115-22. [PubMed]
- Rodriguez F, Langer F, Harrington KB, et al. Effect of cutting second-order chordae on in-vivo anterior mitral leaflet compound curvature. J Heart Valve Dis 2005;14:592-601; discussion 601-2. [PubMed]
- Dreyfus GD, Bahrami T, Alayle N, et al. Repair of anterior leaflet prolapse by papillary muscle repositioning: a new surgical option. Ann Thorac Surg 2001;71:1464-70. [PubMed]
- Godley RW, Wann LS, Rogers EW, et al. Incomplete mitral leaflet closure in patients with papillary muscle dysfunction. Circulation 1981;63:565-71. [PubMed]
- Turi ZG. Cardiology patient page. Mitral valve disease. Circulation 2004;109:e38-41. [PubMed]
- Jain S, Mankad SV. Echocardiographic assessment of mitral stenosis: echocardiographic features of rheumatic mitral stenosis. Cardiol Clin 2013;31:177-91. [PubMed]
- Nichol PM, Gilbert BW, Kisslo JA. Two-dimensional echocardiographic assessment of mitral stenosis. Circulation 1977;55:120-8. [PubMed]
- Silbiger JJ. Anatomy, mechanics, and pathophysiology of the mitral annulus. Am Heart J 2012;164:163-76. [PubMed]
- Baumgartner H, Hung J, Bermejo J, et al. Echocardiographic assessment of valve stenosis: EAE/ASE recommendations for clinical practice. J Am Soc Echocardiogr 2009;22:1-23. [PubMed]
- Bonou M, Vouliotis AI, Lampropoulos K, et al. Continuity equation is the echocardiographic method of choice to assess degenerative mitral stenosis. Cardiol J 2011;18:577-80. [PubMed]
- Payvandi LA, Rigolin VH. Calcific mitral stenosis. Cardiol Clin 2013;31:193-202. [PubMed]
- Iung B, Vahanian A. Epidemiology of valvular heart disease in the adult. Nat Rev Cardiol 2011;8:162-72. [PubMed]
- Freed LA, Levy D, Levine RA, et al. Prevalence and clinical outcome of mitral-valve prolapse. N Engl J Med 1999;341:1-7. [PubMed]
- Anyanwu AC, Adams DH. Etiologic classification of degenerative mitral valve disease: Barlow's disease and fibroelastic deficiency. Semin Thorac Cardiovasc Surg 2007;19:90-6. [PubMed]
- Fornes P, Heudes D, Fuzellier JF, et al. Correlation between clinical and histologic patterns of degenerative mitral valve insufficiency: a histomorphometric study of 130 excised segments. Cardiovasc Pathol 1999;8:81-92. [PubMed]
- Nesta F, Leyne M, Yosefy C, et al. New locus for autosomal dominant mitral valve prolapse on chromosome 13: clinical insights from genetic studies. Circulation 2005;112:2022-30. [PubMed]
- Tsang W, Freed BH, Lang RM. The role of 3-dimensional echocardiography in the diagnosis and management of mitral valve disease: Myxomatous valve disease. Cardiol Clin 2013;31:203-15. [PubMed]
- David TE, Omran A, Armstrong S, et al. Long-term results of mitral valve repair for myxomatous disease with and without chordal replacement with expanded polytetrafluoroethylene sutures. J Thorac Cardiovasc Surg 1998;115:1279-85; discussion 1285-6. [PubMed]
- Ng CM, Cheng A, Myers LA, et al. TGF-beta-dependent pathogenesis of mitral valve prolapse in a mouse model of Marfan syndrome. J Clin Invest 2004;114:1586-92. [PubMed]
- Allen H, Harris A, Leatham A. Significance and prognosis of an isolated late systolic murmur: a 9- to 22-yearyearfollow-up. Br Heart J 1974;36:525-32. [PubMed]
- Angelini A, Ho SY, Anderson RH, et al. Disjunction of the mitral annulus in floppy mitral valve. N Engl J Med 1988;318:188-9. [PubMed]
- Barlow JB, Bosman CK. Aneurysmal protrusion of the posterior leaflet of the mitral valve. An auscultatory-electrocardiographic syndrome. Am Heart J 1966;71:166-78. [PubMed]
- Barber JE, Kasper FK, Ratliff NB, et al. Mechanical properties of myxomatous mitral valves. J Thorac Cardiovasc Surg 2001;122:955-62. [PubMed]
- Chou HT, Shi YR, Hsu Y, et al. Association between fibrillin-1 gene exon 15 and 27 polymorphisms and risk of mitral valve prolapse. J Heart Valve Dis 2003;12:475-81. [PubMed]
- Grande-Allen KJ, Borowski AG, Troughton RW, et al. Apparently normal mitral valves in patients with heart failure demonstrate biochemical and structural derangements: An extracellular matrix and echocardiographic study. J Am Coll Cardiol 2005;45:54-61. [PubMed]
- Rabkin E, Aikawa M, Stone JR, et al. Activated interstitial myofibroblasts express catabolic enzymes and mediate matrix remodeling in myxomatous heart valves. Circulation 2001;104:2525-32. [PubMed]
- Barlow JB, Pocock WA. Billowing, floppy, prolapsed or flail mitral valves? Am J Cardiol 1985;55:501-2. [PubMed]
- St John Sutton M, Weyman AE. Mitral valve prolapse prevalence and complications: an ongoing dialogue. Circulation 2002;106:1305-7. [PubMed]
- Horstkotte D, Schulte HD, Niehues R, et al. Diagnostic and therapeutic considerations in acute, severe mitral regurgitation: experience in 42 consecutive patients entering the intensive care unit with pulmonary edema. J Heart Valve Dis 1993;2:512-22. [PubMed]
- Hueb AC, Jatene FB, Moreira LF, et al. Pomerantzeff PM, Kallas E, de Oliveira SA. Ventricular remodeling and mitral valve modifications in dilated cardiomyopathy: new insights from anatomic study. J Thorac Cardiovasc Surg 2002;124:1216-24. [PubMed]
- McCully RB, Enriquez-Sarano M, Tajik AJ, et al. Overestimation of severity of ischemic/functional mitral regurgitation by color Doppler jet area. Am J Cardiol 1994;74:790-3. [PubMed]
- Magne J, Girerd N, Senechal M, et al. Mitral repair versus replacement for ischemic mitral regurgitation: comparison of short-term and long-term survival. Circulation 2009;120:S104-11. [PubMed]
- Watanabe N, Ogasawara Y, Yamaura Y, et al. Geometric differences of the mitral valve tenting between anterior and inferior myocardial infarction with significant ischemic mitral regurgitation: quantitation by novel software system with transthoracic real-time three-dimensional echocardiography. J Am Soc Echocardiogr 2006;19:71-5. [PubMed]
- Glower DD, Tuttle RH, Shaw LK, et al. Patient survival characteristics after routine mitral valve repair for ischemic mitral regurgitation. J Thorac Cardiovasc Surg 2005;129:860-8. [PubMed]