Systematic review of robotic minimally invasive mitral valve surgery
Introduction
Minimally invasive mitral valve surgery (MVS) has been proven to be an effective alternative to a conventional sternotomy approach, with both low perioperative mortality and morbidity, and a long term durability comparable to conventional techniques (1). However minimally invasive approaches presented unique challenges to surgeons, including reduced intrathoracic space, difficult visualization of the valve and sub-valvular apparatus, and long-shafted instrumentation with limited dexterity, which triggered the development of robotic-assistance devices. The first was the AESOP3000 (Computer Motion, Santa Barbara, CA), a voice-controlled robotic arm with a videoscope, designed to provide stable visualization and allow solo operations (2).
The next major evolution was the development of robotic telemanipulators, which overcame the limitation of long-shafted instruments by providing 3-dimensional vision and articulating instruments with a range of motion similar to the human hand. Carpentier and Mohr independently performed the first successful robotic MVS cases in 1998 using prototypes of the da Vinci Surgical System (Intuitive Surgical Inc., Sunnyvale, California, USA) telemanipulator (3,4). Since then, telemanipulators have continued to evolve and the latest systems provide high resolution 3D visualization, up to 10x magnification of the operating field, movement scaling, and dual console systems for surgeon co-operation and training (5). The purpose of this systematic review was to assess the clinical outcomes and cost-effectiveness of robotic telemanipulator-assisted MVS.
Methods
Literature search strategy
Electronic searches were performed on Ovid MEDLINE, EMBASE, Cochrane Central Register of Controlled Trials (CCTR), Cochrane Database of Systematic Reviews (CDSR), ACP Journal Club and Database of Abstracts of Review of Effectiveness (DARE) from their dates of inception to August 2013. The search strategy included a combination of ‘robotic’ or ‘telemanipulator’ or ‘computer-assisted’ and ‘mitral’ as keywords and MeSH headings. The reference lists of all retrieved articles were reviewed for further identification of potentially relevant studies. All relevant articles identified were assessed with application of the predefined selection criteria.
Selection criteria
Studies that reported clinical outcomes, cost-analysis or learning curve analysis of robotic MVS, including mitral valve repair and replacement, were selected for qualitative analysis. When institutions published duplicate trials, only the most updated reports were included for qualitative appraisal. Studies with overlapping patient populations but analysing different endpoints were included in qualitative review. All publications were limited to human subjects and English language. Abstracts, case reports, conference presentations, editorials and expert opinions were excluded.
Critical appraisal
The quality of the evidence from each study was assessed using the GRADE system (6). Data was extracted from texts, tables and figures of selected studies. When insufficient or ambiguous data were presented from publications, corresponding authors were contacted to provide additional information. Discrepancies between the two investigators were resolved by discussion and consensus with senior investigators (T.D.Y and F.M).
Results
Included trials and quality of evidence
Search methods identified 61 potentially relevant papers. Reasons for exclusion are detailed in Figure 1. Nine studies were excluded because results were included in later studies with cumulative patients, cumulative follow-up, or more detailed analyses (4,7-14). Studies that had overlapping patient populations but analyzed different variables (for example specific surgical techniques or cost analysis) were included. Thus 27 studies were included in the qualitative appraisal (15-18). Sixteen studies that had ≥50 patients were included in quantitative appraisal to improve the reliability of the data presented (10,19-33) and their study characteristics are presented in Table 1.
All studies were observational in nature, and thus the quality of evidence is limited. The quality of evidence according to the GRADE system (on a scale of + to ++++ confidence) is reported for each study in Table 1 (6). Studies rated +++ indicate that reviewers had a moderate level of confidence in the effect estimate, but there may be a possibility that it is substantially different. One study was a 10-centre phase II clinical trial in the USA (24). Most studies were single-institution and since studies were restricted to those with ≥50 patients, the number of institutions was limited.
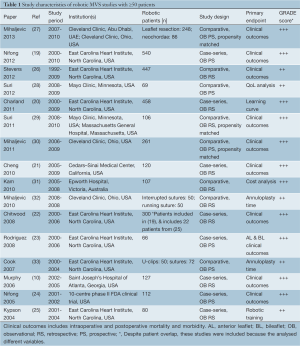
Full table
Baseline characteristics
Table 1 demonstrates the range of MV pathologies, primary repair techniques used, and preoperative patient characteristics. Most retrospective studies had excluded patients with heavily calcified MV annuli, ischaemic disease, rheumatic disease, bacterial endocarditis, and those requiring MV replacement in some studies. Other reasons for exclusion included severe pulmonary hypertension and requirement for coronary artery bypass grafting. Robotic patients were relatively young, with mean ages ranging from 52.6-58.4 years. Patients had high left ventricular ejection fractions (LVEF), with all studies reporting mean ejection fractions >56%. Patients were also relatively asymptomatic, with most studies reporting
Intraoperative and postoperative outcomes
The intraoperative outcomes reported by studies with ≥50 patients are presented in Table 2, and the short term postoperative outcomes in Table 3. Rate of conversion to non-robotic MVS through mini-thoracotomy or sternotomy ranged from 0.0-9.1%. Reasons for conversion included da Vinci system malfunction and external instrument conflicts, atherosclerotic femoral vessels precluding safe peripheral cannulation, poor surgical exposure, bleeding, inability to arrest the heart, repair failure and the need for MV replacement (22,30).
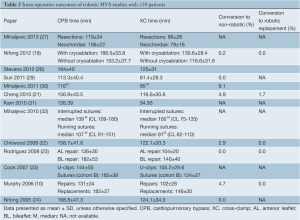
Full table
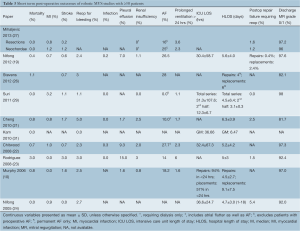
Full table
The most frequently performed concomitant robotic procedures were ablation for atrial fibrillation (AF) (up to 24.2%), left atrial appendage (LAA) occlusion (up to 12.5%), closure of atrial septal defects (ASD) and patent foramen ovale (PFO) (up to 13%), and tricuspid valve repair was also possible (Table 4).
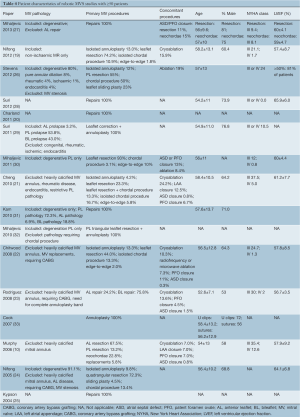
Full table
Mean cardiopulmonary bypass (CPB) times ranged from 106±22 to 188.5±53.8 min and mean cross-clamp (XC) times ranged from 79±16 to 140±40 min (Table 2). There was only one study that reported CPB and XC times separately for MVS with or without cryoablation for AF: CPB time was 188.5±53.8 min with cryoablation, and 153.2±37.7 min without cryoablation; and XC time was 130.6±28.4 min with cryoablation, and 116.6±31.6 min without cryoablation (19). There was one study that reported CPB and XC times for anterior leaflet (AL) and bileaflet (BL) repairs specifically: CPB was 136±30 min for AL repair, and 182±53 min for BL repair; and XC time was 104±20 min for AL repair, and 140±40 min for BL repairs (23). There were no studies with ≥50 patients reporting mean CPB and XC times for robotic MV replacement; the range for studies with 10,34).
Early mortality was in the range of 0.0-3.0%, with the majority of case-series reporting 48 hours) (Table 3). The rate of early post-operative failure of repair requiring reoperation ranged from 1.5-5.4%. Postoperative transthoracic echocardiography prior to discharge demonstrated that 81.7-97.6% of patients had no or only trace amounts of mitral regurgitation (MR).
The mean intensive care unit length of stay (ICU LOS) ranged from 12.3±6.7 to 36.6±24.7 hours. The mean hospital length of stay (HLOS) ranged from 3.1±0.3 to 6.3±3.9 days.
Intermediate and long-term outcomes
The quantity of evidence for intermediate and long-term outcomes of robotic MVS was limited. The following preoperative variables were identified as independent predictors of late mortality: age (65-75 years: HR 2.63), valvular disease of ischaemic (HR 1.92) and rheumatic (HR 2.10) aetiology, renal failure (HR 4.77), NYHA class III-IV (HR 1.68), LVEF (40-50%: HR 1.75), and prior valve operation (HR 3.59) (26). Robotic surgical approach did not predict late-mortality (HR of 0.72, P=0.229) compared to sternotomy and videoscopic MVS.
Four studies reported intermediate to long-term echocardiographic follow-up (10,21-23). Chitwood and colleagues’ study reported at a mean follow-up time of 815±459 days (n=279) that 68.8% had no or trace MR, 23.6% had mild MR, 5.3% had moderate MR and 2.2% had severe MR (22). Chitwood′s group also presented a study of AL and BL repairs, reported at a mean follow-up time of 609±436 days (n=60), that 58.3% had no or trace MR, 32% had mild MR, 3% had moderate MR and 6.7% had severe MR (23). Cheng and colleagues’ study reported at a mean follow-up 373±332 days (n=107) that 62.6% had no or trace MR, 26.2% had mild MR, 8.4% had moderate MR, and 2.8% had severe MR (21). Murphy and colleagues’ study reported at a mean follow-up 8.4±8.1 months (n=98) that 88.8% had grade 0 MR, 8.2% had 1+ MR and 3.1% had 2+ MR (10). One study reported NYHA functional class, and found at mean follow-up of 13.7±8.9 months that 91.6% were class I, 6.7% were class II, 1.7% were class III and 0.0% were class IV (10).
One study reported 5-year Kaplan-Meier survival and freedom from reoperation, which was 96.6%±1.5% and 93.8%±1.6%, respectively (22). The total reoperation rate was 5.3% and the mean time to reoperation was 319±327 days (15-946 days). In a later study which included these patients, the reoperation rate had decreased to 2.9% and the mean time to reoperation was 303±281 days (15-946 days) (19).
Discussion
Specific robotic repair techniques
All types of MV repair and replacement were possible using the telemanipulator (7,35). AL and BL prolapse are usually more difficult to repair than posterior leaflet prolapse, requiring more advanced techniques with less tolerance for error, and thus have been associated with increased reoperation rates (36). Rodriguez and colleagues’ study examined robotic AL and BL repair specifically, which reported a 9% rate of reoperation at a mean follow-up of two years (23). Since other non-robotic AL and BL studies have reported similar times to reoperation that then plateau, the study concluded that a similar course would apply to their cohort (37).
Neochordal repair techniques are an option in patients with extensive posterior leaflet prolapse where resection could compromise repair success (38). Mihaljevic and colleagues’ propensity matched comparison of neochordal vs. resectional techniques for robotic posterior mitral leaflet repair in degenerative disease found that the number of intraoperative attempts required to achieve satisfactory repair was similar in both groups (single attempt required in 91% vs. 91%, two attempts in 9.3% vs. 9.3%), and the degree of residual MR was also similar (27). There were no differences in postoperative mortality or morbidities. The robotic technology also allowed the surgeon superior visualization of the subvalvular apparatus, helping to decrease the technical difficulty of the neochordal technique. Chu and colleagues have also described a hair-cut technique of isolated posterior leaflet repair, which involves resecting the prolapsing margin of a tall P2 to reduce it′s height and transferring secondary chords either from P2 or the AL (34). This technique aims to preserve the tissue and physiologic mobility of the posterior leaflet, taking the plane of resection of excess tissue from the leaflet-annular junction to the free edge thus reducing the number of suture lines and simplifying the repair. In their 17 patient series there were no postoperative mortalities or morbidities, and only mild postoperative MR in one case.
Increased time required to repair or replace the valve resulting in longer CPB and XC times remains the major drawback of a robotic approach. East Carolina University developed a double-arm U-clip made of nitinol alloy (allowing a deployed clip to return to its preformed shape) to secure an annuloplasty band, and designed to be faster than knot tying to reduce operating times. When compared to sutures for securing a flexible posterior annuloplasty band, the U-clips resulted in a shorter total time required to secure the band (101±45 vs. 169±68 sec, P33). There was one failed repair (2%), due to separation of U-clips from the mitral annular tissue but still attached to the annuloplasty band. Smith and colleagues have also described using single-armed U-clips to secure premeasured neochordae for leaflet prolapse, which helped decrease their XC and CPB times (39). Recently, Nifong and colleagues also presented preliminary results of the novel Cor-Knot device (LSI Solutions, Inc., Victor, NY), which uses a titanium clip to secure sutures and eliminate knot tying (40). When compared to robotic knot tying (n=288), the Cor-Knot (n=48) significantly reduced time for securing sutures (48.6±24.6 vs. 72.6±36.0 sec, Pvs. 36.6±10.2 min, Pvs. 160.3±40.1 min, Pvs. 123.0±33.3 min, Pvs. interrupted sutures for securing an annuloplasty band, which also resulted in a significantly shorter operating time (38 min less, P=0.01), CPB time (32 min less, P=0.0003) and XC time (19 minutes less, P=0.0008), without compromising rates of in-hospital mortality, repair failure or post-operative MR grades (32). They reported that using two running sutures and one anchoring suture provided the optimal balance of fixation and steps required.
Concomitant ablation for AF
Up to 50% of patients undergoing MVS have AF, and thus combining ablative treatment at the time of robotic MVS is ideal (38,41). Reade and colleagues initially reported using a flexible microwave catheter to ablate the peri-pulmonary vein region concomitantly with robotic MVS, and found prolonged operating times but a positive risk-benefit ratio (42). Nifong and colleagues described their experience in 86 AF patients (48.8% paroxysmal, 51.2% persistent) undergoing the Cox-Maze III cryoablative procedure, currently considered the gold standard for AF surgery, concomitantly with robotic MVS (15.4% of their total robotic MVS cohort) (19). In their follow-up period of 351±281 days there was 96.5% freedom from AF, demonstrating the procedure′s efficacy. Compared to robotic MVS alone, the combined cryoablative patients were significantly older (65.6±10.8 vs. 56.1±12.9, Pvs. 153.2±37.7 min, Pvs. 116.6±31.6 min, Pvs. 0.2%). The study concluded that combining the two procedures provides results similar to conventional techniques.
Cost analysis
Two studies performed cost analysis of their robotic MIMVS programs (31,43). Morgan and colleagues retrospectively reviewed 20 robotic MIMVS (and 20 robotic ASD closures) compared to sternotomy MVS at Columbia University, New York, USA in 2005 (43). The most significant intraoperative drivers of robotic MVS cost (total US$9,507±1,598) were supplies and operating room time, the latter of which decreases as surgeons overcome their learning curve. Supplies included the robotic instruments, which can only be used a maximum of ten times before being disposed. The main postoperative drivers of cost (total US$4,387±1,690) were length of stay in ICU and room costs, both of which were lower in robotic MVS compared to sternotomy MVS, but not statistically significant. Thus they found that robotic technology did not significantly increase total hospital cost compared to sternotomy MVS (US$13,894±2,774 vs. US$14,538±1,697, P=0.539). There was however an initial capital investment in the robotic technology by their institution of approximately US$1,000,000 and annual operating fees of US$100,000 after the 1st year, that when amortised into the cost (at a rate of US$2,800 per case, calculated by assuming 100 cases/yr for 5 yrs) resulted in robotic procedures becoming more expensive than sternotomy procedures. Kam and colleagues performed an analysis of robotic MVS compared to conventional MVS at Epworth Hospital, Melbourne, Australia in 2010 (31). They similarly found that higher mean intraoperative costs (AU$12,328 vs. AU$9,755, no P value) were offset by lower postoperative costs (AU$6,174 vs. AU$8,124, Pvs. AU$17,879.80, average difference AU$623, 95% CI: ?$282 to $1,529, P=0.176)
QoL
Improvement in postoperative QoL and expeditious return to work are primary objectives of minimally-invasive surgery. Suri and colleagues performed a comprehensive survey-based QoL analysis into this topic, comparing robotic (n=69) to sternotomy MVS (n=202) (28). In the 0-12 months postoperative period, robotic MVS resulted in better Duke Activity Status Index scores (55.1±2.1 vs. 45.1±2.5, P=0.003, i.e., a moderate difference), better Short Form 12-Item Health Survey scores in the physical domain (55.4±1.2 vs. 48.0±1.4, Pvs. 1.7±0.3, P=0.014), severity (0.4±0.3 vs. 1.7±0.3, P=0.006), and fatigue (2.3±0.4 vs. 4.3±0.5, P=0.003). In the 12-24 month postoperative period the only significant difference was a better Linear Analogue Self-Assessment overall QoL score for robotic MVS (9.3±0.2 vs. 8.6±0.2, P=0.034). The median time to return to work was also lower for robotic MVS (33 vs. 54 days, P=0.001). The individual baseline characteristics between the robotic and sternotomy groups were similar, but the overall age-weighted Charlson score was higher in the sternotomy group, so the authors performed an adjusted analysis that found similar results. The authors recognized a number of limitations, including the observational retrospective nature of the study, no preoperative QoL assessment and the limitations associated with survey-based research. More studies with larger patient populations and more time intervals are justified. Mihaljevic and colleagues also analyzed the immediate postoperative pain scores of their propensity matched comparison of robot to sternotomy MVS (n=106). They found that pain scores were similar, with 70% of both groups reporting no or little pain by the fourth postoperative day (30). The authors postulated that the relatively small patient population and stringent pain management might have masked any difference.
Training and learning curve
Robotic telemanipulator-based surgery represents a significant change from current techniques, and thus an examination of learning curves and training programs is important in achieving optimal performance. Charland and colleagues identified seven variables in 500 operations that significantly affected total robot time (dock-in to dock-out) (coefficient represented in brackets; positive values increase time taken): the presence of a fellow (0.0660), annuloplasty band size (0.0119), the log of the number of patients (C0.0709), the use of nitinol U-clips for band insertion (C0.1148), the inclusion of a chordal procedure in the repair (0.0437), performing a concomitant AF ablation (0.0476), and leaflet resections (0.0562) (all P20). Another factor contributing to the learning curve described by Rodriguez and colleagues was the lack of tactile feedback during suturing (23). This necessitates the use of tissue displacement and deformation as visual cues to the suture depth and tension, for which there is evidence that this hinders novice surgeons, but not more experienced surgeons (44).
In the multicentre phase II clinical trial of robotic mitral repair CPB times decreased by 4.3 minutes per progressive case, XC times by 3.7 minutes per case, and operative times by 4.4 minutes per case (24). They found that times began to decrease after the surgeon had performed approximately 15 cases. The trainee surgeon training program involved sequentially: a didactic overview of surgical robotics, inanimate laboratory training, animal laboratory training, cadaver laboratory training, live case observation (11,24).
Cheng and colleagues divided their cohort of 120 patients into those operated on with the first generation da Vinci robot (n=74) or the second generation da Vinci Si HD (n=46) (21). The da Vinci Si HD added improved 3D vision and a 4th robotic arm allowing attachment of an adjustable atrial retractor to enhance exposure and thus suturing accuracy. All of their failed repairs (5/74) occurred with the older da Vinci robot, and there were no major complications and reoperation for postoperative bleeding of 1.7% with the newer da Vinci Si HD. Since the two groups were in chronological order, it is difficult to separate any improvements in clinical outcomes due to improved robotics or increased experience. Similarly, Suri and colleagues found that CPB (131.2±45.0 vs. 95.9±25.6 min, Pvs. 68.7±19.1 min, Pvs. 1.6±3.4 hrs, Pvs. 12.3±6.7 hrs, Pvs. 3.1±0.3 days, P29).
Limitations
All studies were observational and either prospective or retrospective in nature, and thus any conclusions that can be drawn are limited. There have also been multiple generations of robotic telemanipulators (da Vinci, da Vinci Si HD) with potential improvements in latter designs that may have reduced procedural times and improved clinical outcomes. Studies have shown that results are reproducible, but published results still originated from a limited number of highly specialised institutions with significant experience in the development and training of robotic MVS.
Conclusions
Patients undergoing robotic MVS most commonly have degenerative MV disease, are generally low risk, and have good left ventricular function. All subtypes of mitral valve prolapse are repairable with robotic techniques. Bearing in mind the limitations of our review, and of the included studies, the overall rates of early postoperative mortality and morbidity are low. CPB and XC times are longer in robotic MVS compared to sternotomy, and novel techniques such as the Cor-Knot, Nitinol clips or running sutures may reduce the time required. Improvements in postoperative QoL and expeditious return to work offset the increase in equipment and intraoperative cost. Evidence for long-term outcomes is as yet limited.
Acknowledgements
There has been no financial assistance with the project.
Disclosure: The authors declare no conflict of interest.
References
- McClure RS, Cohn LH, Wiegerinck E, et al. Early and late outcomes in minimally invasive mitral valve repair: an eleven-year experience in 707 patients. J Thorac Cardiovasc Surg 2009;137:70-5. [PubMed]
- Mohr FW, Onnasch JF, Falk V, et al. The evolution of minimally invasive valve surgery--2 year experience. Eur J Cardiothorac Surg 1999;15:233-8; discussion 238-9. [PubMed]
- Carpentier A, Loulmet D, Aupcle B, et al. Computer assisted open heart surgery. First case operated on with success. C R Acad Sci III 1998;321:437-42. [PubMed]
- Falk V, Autschbach R, Krakor R, et al. Computer-enhanced mitral valve surgery: toward a total endoscopic procedure. Semin Thorac Cardiovasc Surg 1999;11:244-9. [PubMed]
- Lehr EJ, Rodriguez E, Chitwood WR. Robotic cardiac surgery. Curr Opin Anaesthesiol 2011;24:77-85. [PubMed]
- Balshem H, Helfand M, Schnemann HJ, et al. GRADE guidelines: 3. Rating the quality of evidence. J Clin Epidemiol 2011;64:401-6. [PubMed]
- Suri RM, Burkhart HM, Rehfeldt KH, et al. Robotic mitral valve repair for all categories of leaflet prolapse: improving patient appeal and advancing standard of care. Mayo Clin Proc 2011;86:838-44. [PubMed]
- Tatooles AJ, Pappas PS, Gordon PJ, et al. Minimally invasive mitral valve repair using the da Vinci robotic system. Ann Thorac Surg 2004;77:1978-82; discussion 1982-4.
- Sobieski MA 2nd, Slaughter MS, Hart DE, et al. Peripheral cardiopulmonary bypass with modified assisted venous drainage and transthoracic aortic crossclamp: optimal management for robotic mitral valve repair. Perfusion 2003;18:307-11. [PubMed]
- Murphy DA, Miller JS, Langford DA, et al. Endoscopic robotic mitral valve surgery. J Thorac Cardiovasc Surg 2006;132:776-81. [PubMed]
- Chitwood WR Jr, Nifong LW, Chapman WH, et al. Robotic surgical training in an academic institution. Ann Surg 2001;234:475-84; discussion 484-6. [PubMed]
- Autschbach R, Onnasch JF, Falk V, et al. The Leipzig experience with robotic valve surgery. J Card Surg 2000;15:82-7. [PubMed]
- Morgan JA, Argenziano M, Smith CR. Robotic valve surgery: how does the future look? Adv Cardiol 2004;41:157-63. [PubMed]
- Chitwood WR Jr. Current status of endoscopic and robotic mitral valve surgery. Ann Thorac Surg 2005;79:S2248-53. [PubMed]
- Smith JM, Stein H, Engel AM, et al. Totally endoscopic mitral valve repair using a robotic-controlled atrial retractor. Ann Thorac Surg 2007;84:633-7. [PubMed]
- McClure RS, Kiaii B, Novick RJ, et al. Computer-enhanced telemanipulation in mitral valve repair: preliminary experience in Canada with the da Vinci robotic system. Can J Surg 2006;49:193-6. [PubMed]
- Jones BA, Krueger S, Howell D, et al. Robotic mitral valve repair: a community hospital experience. Tex Heart Inst J 2005;32:143-6. [PubMed]
- Mohr FW, Falk V, Diegeler A, et al. Computer-enhanced robotic cardiac surgery: experience in 148 patients. J Thorac Cardiovasc Surg 2001;121:842-53. [PubMed]
- Nifong LW, Rodriguez E, Chitwood WR Jr. 540 consecutive robotic mitral valve repairs including concomitant atrial fibrillation cryoablation. Ann Thorac Surg 2012;94:38-42; discussion 43. [PubMed]
- Charland PJ, Robbins T, Rodriguez E, et al. Learning curve analysis of mitral valve repair using telemanipulative technology. J Thorac Cardiovasc Surg 2011;142:404-10. [PubMed]
- Cheng W, Fontana GP, De Robertis MA, et al. Is robotic mitral valve repair a reproducible approach? J Thorac Cardiovasc Surg 2010;139:628-33. [PubMed]
- Chitwood WR Jr, Rodriguez E, Chu MW, et al. Robotic mitral valve repairs in 300 patients: a single-center experience. J Thorac Cardiovasc Surg 2008;136:436-41. [PubMed]
- Rodriguez E, Nifong LW, Chu MW, et al. Robotic mitral valve repair for anterior leaflet and bileaflet prolapse. Ann Thorac Surg 2008;85:438-44; discussion 444. [PubMed]
- Nifong LW, Chitwood WR, Pappas PS, et al. Robotic mitral valve surgery: a United States multicenter trial. J Thorac Cardiovasc Surg 2005;129:1395-404. [PubMed]
- Kypson AP, Nifong LW, Chitwood WR Jr. Robot-assisted surgery: training and re-training surgeons. Int J Med Robot 2004;1:70-6. [PubMed]
- Stevens LM, Rodriguez E, Lehr EJ, et al. Impact of timing and surgical approach on outcomes after mitral valve regurgitation operations. Ann Thorac Surg 2012;93:1462-8. [PubMed]
- Mihaljevic T, Pattakos G, Gillinov AM, et al. Robotic posterior mitral leaflet repair: neochordal versus resectional techniques. Ann Thorac Surg 2013;95:787-94. [PubMed]
- Suri RM, Antiel RM, Burkhart HM, et al. Quality of Life after early mitral valve repair using conventional and robotic approaches. Ann Thorac Surg 2012;93:761-9. [PubMed]
- Suri RM, Burkhart HM, Daly RC, et al. Robotic mitral valve repair for all prolapse subsets using techniques identical to open valvuloplasty: establishing the benchmark against which percutaneous interventions should be judged. J Thorac Cardiovasc Surg 2011;142:970-9. [PubMed]
- Mihaljevic T, Jarrett CM, Gillinov AM, et al. Robotic repair of posterior mitral valve prolapse versus conventional approaches: potential realized. J Thorac Cardiovasc Surg 2011;141:72-80.e1-4.
- Kam JK, Cooray SD, Kam JK, et al. A cost-analysis study of robotic versus conventional mitral valve repair. Heart Lung Circ 2010;19:413-8. [PubMed]
- Mihaljevic T, Jarrett CM, Gillinov AM, et al. A novel running annuloplasty suture technique for robotically assisted mitral valve repair. J Thorac Cardiovasc Surg 2010;139:1343-4. [PubMed]
- Cook RC, Nifong LW, Enterkin JE, et al. Significant reduction in annuloplasty operative time with the use of nitinol clips in robotically assisted mitral valve repair. J Thorac Cardiovasc Surg 2007;133:1264-7. [PubMed]
- Chu MW, Gersch KA, Rodriguez E, et al. Robotic haircut mitral valve repair: posterior leaflet-plasty. Ann Thorac Surg 2008;85:1460-2. [PubMed]
- Gao C, Yang M, Xiao C, et al. Robotically assisted mitral valve replacement. J Thorac Cardiovasc Surg 2012;143:S64-7. [PubMed]
- David TE, Ivanov J, Armstrong S, et al. A comparison of outcomes of mitral valve repair for degenerative disease with posterior, anterior, and bileaflet prolapse. J Thorac Cardiovasc Surg 2005;130:1242-9. [PubMed]
- Gillinov AM, Cosgrove DM, Lytle BW, et al. Reoperation for failure of mitral valve repair. J Thorac Cardiovasc Surg 1997;113:467-73; discussion 473-5. [PubMed]
- Ha?ssaguerre M, Ja?s P, Shah DC, et al. Spontaneous initiation of atrial fibrillation by ectopic beats originating in the pulmonary veins. N Engl J Med 1998;339:659-66. [PubMed]
- Smith JM, Stein H. Endoscopic placement of multiple artificial chordae with robotic assistance and nitinol clip fixation. J Thorac Cardiovasc Surg 2008;135:610-4. [PubMed]
- Nifong LW, Alwair H, Parker D, et al. Significant Reduction in Operative Times Using Cor-Knot? In Robot-Assisted Mitral Valve Repair. International Society for Minimally Invasive Cardiothoracic Surgery 2013:1.
- Gillinov AM. Ablation of atrial fibrillation with mitral valve surgery. Curr Opin Cardiol 2005;20:107-14. [PubMed]
- Reade CC, Johnson JO, Bolotin G, et al. Combining robotic mitral valve repair and microwave atrial fibrillation ablation: techniques and initial results. Ann Thorac Surg 2005;79:480-4. [PubMed]
- Morgan JA, Thornton BA, Peacock JC, et al. Does robotic technology make minimally invasive cardiac surgery too expensive? A hospital cost analysis of robotic and conventional techniques. J Card Surg 2005;20:246-51. [PubMed]
- Reiley CE, Akinbiyi T, Burschka D, et al. Effects of visual force feedback on robot-assisted surgical task performance. J Thorac Cardiovasc Surg 2008;135:196-202. [PubMed]